A Process With A Calculated Positive Q
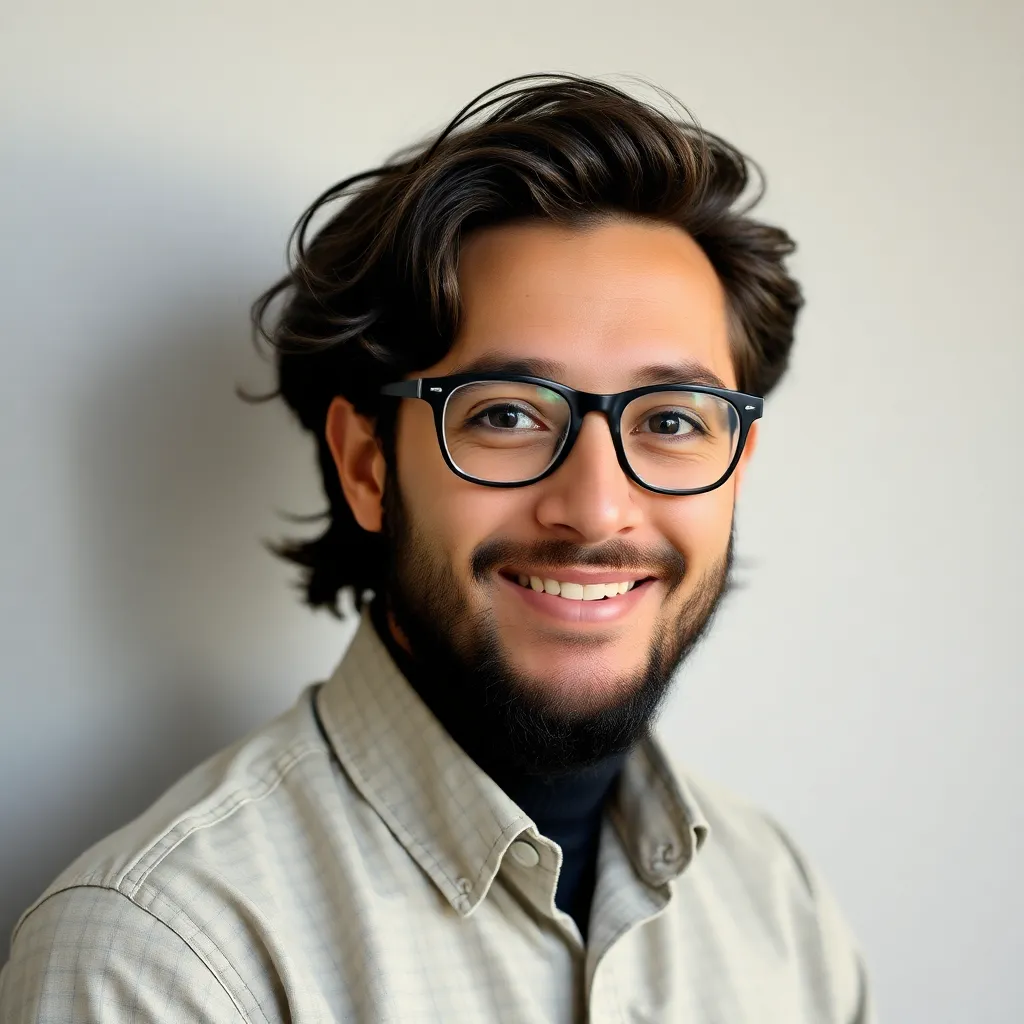
Breaking News Today
Apr 22, 2025 · 5 min read

Table of Contents
A Process with a Calculated Positive Q: Understanding and Achieving Thermodynamic Favorability
The concept of a positive Q, or more precisely, a positive change in enthalpy (ΔH), is fundamental in thermodynamics and process engineering. It signifies a process that absorbs heat from its surroundings, often referred to as an endothermic process. While a negative ΔH (exothermic process, releasing heat) is often perceived as more favorable, many crucial industrial processes and natural phenomena rely on endothermic reactions with carefully calculated positive Q values. This article delves into the intricacies of processes with calculated positive Q, exploring their characteristics, applications, and the strategies employed to ensure their thermodynamic favorability and efficiency.
Understanding Enthalpy and its Role in Process Design
Before diving into processes with a calculated positive Q, let's establish a clear understanding of enthalpy. Enthalpy (H) is a thermodynamic property representing the total heat content of a system at constant pressure. The change in enthalpy (ΔH) during a process reflects the heat exchanged between the system and its surroundings. A positive ΔH indicates that the system absorbs heat, while a negative ΔH signifies that the system releases heat.
ΔH = H<sub>products</sub> - H<sub>reactants</sub>
The significance of ΔH lies in its predictive power regarding the spontaneity of a reaction under constant pressure. However, ΔH alone doesn't fully determine spontaneity. The Gibbs Free Energy (ΔG) provides a more complete picture, incorporating both enthalpy and entropy (ΔS).
ΔG = ΔH - TΔS
Where T is the absolute temperature. A negative ΔG indicates a spontaneous process, while a positive ΔG signifies a non-spontaneous process.
Processes with a Calculated Positive Q: Why and How?
Many processes, despite having a positive ΔH, are crucial and feasible. This is often achieved by manipulating the entropy term (TΔS) in the Gibbs Free Energy equation. If the increase in entropy (ΔS) is sufficiently large and the temperature is high enough, the TΔS term can outweigh the positive ΔH, resulting in a negative ΔG and a spontaneous process.
Examples of Processes with Positive Q:
-
Melting of Ice: The transition from ice to water is endothermic (positive ΔH). Heat is absorbed to break the hydrogen bonds holding the water molecules in a rigid lattice. However, the increase in entropy (greater disorder in the liquid state) makes this process spontaneous above 0°C.
-
Photosynthesis: This vital process in plants involves the conversion of carbon dioxide and water into glucose and oxygen. It requires the absorption of light energy (positive ΔH), yet it's driven by the increase in entropy and the input of solar energy.
-
Ammonia Production (Haber-Bosch Process): While the overall reaction is exothermic, certain steps within the complex process involve endothermic reactions. The manipulation of temperature and pressure allows for a favorable equilibrium to produce ammonia.
-
Electrolysis: This process uses electrical energy to drive non-spontaneous chemical reactions, often with a positive ΔH. The input of electrical energy compensates for the endothermic nature of the reaction.
-
Cracking of Hydrocarbons: In the petroleum industry, long-chain hydrocarbons are broken down into smaller, more valuable molecules. This process is endothermic and requires significant heat input. The economic benefits outweigh the energy cost.
Strategies for Achieving Favorable Equilibrium in Endothermic Processes:
Several strategies are employed to enhance the efficiency and spontaneity of processes with a positive ΔH:
1. Temperature Control:
Increasing the temperature favors endothermic reactions. According to Le Chatelier's principle, increasing the temperature shifts the equilibrium towards the product side for endothermic reactions. However, finding the optimal temperature is crucial. Too high a temperature might lead to unwanted side reactions or energy losses.
2. Pressure Control:
Pressure manipulation is less effective for endothermic reactions compared to exothermic ones. The effect depends on the change in the number of gas moles during the reaction. Increasing pressure might favor the side with fewer gas moles.
3. Catalyst Utilization:
Catalysts significantly accelerate the rate of reaction without being consumed themselves. They lower the activation energy, making the reaction proceed faster at a lower temperature, improving efficiency and potentially reducing energy costs.
4. Optimization of Reaction Conditions:
Precise control over reaction parameters such as concentration of reactants, flow rates, and mixing efficiency can significantly impact the reaction yield and overall process efficiency.
5. Coupling with Exothermic Reactions:
Endothermic processes can be coupled with exothermic reactions to achieve overall energy efficiency. The heat released by the exothermic reaction can be used to supply the heat required for the endothermic reaction, reducing the external energy input needed.
Calculating and Analyzing Positive Q in Real-World Applications:
Calculating the enthalpy change (ΔH) for a given process often involves using standard enthalpy of formation values for the reactants and products. These values represent the enthalpy change when one mole of a compound is formed from its constituent elements in their standard states.
ΔH<sub>reaction</sub> = Σ ΔH<sub>f</sub><sup>°</sup>(products) - Σ ΔH<sub>f</sub><sup>°</sup>(reactants)
Sophisticated software and computational tools are often used to model complex reactions and predict the behavior under different conditions. This allows engineers to optimize the process for maximum efficiency and minimize energy consumption and waste.
Economic Considerations and Sustainability:
While processes with a positive Q require energy input, their economic viability depends on several factors:
-
Value of the Products: If the products are high-value chemicals, the energy cost of the endothermic process might be justified.
-
Energy Source: Utilizing renewable energy sources to power the endothermic process contributes to sustainability and reduces the overall environmental impact.
-
Process Optimization: Efficient process design and operation minimize energy waste and maximize product yield.
-
Waste Management: Effective waste management strategies minimize environmental pollution and contribute to sustainable practices.
Conclusion:
Processes with a calculated positive Q, while requiring energy input, play a critical role in various industries and natural phenomena. A comprehensive understanding of thermodynamics, coupled with strategic process design and optimization, is essential to harness the potential of these endothermic reactions. By carefully controlling temperature, pressure, utilizing catalysts, and possibly coupling with exothermic processes, it's possible to achieve favorable equilibrium and render these processes economically viable and environmentally sustainable. The ongoing development of efficient and environmentally friendly technologies will further expand the application of processes with calculated positive Q values in the future. Further research into catalyst development, process intensification, and renewable energy integration promises even greater efficiency and sustainability in these vital processes.
Latest Posts
Latest Posts
-
The Vast Majority Of Crime In Texas Involves
Apr 22, 2025
-
The First Personnel Recovery Pr Task Is
Apr 22, 2025
-
How Should Hunters Who Are Hunting Together Walk
Apr 22, 2025
-
Which Of The Following Gases Is Not A Greenhouse Gas
Apr 22, 2025
-
Person Centered Planning Teams Are The Same As Iep Teams
Apr 22, 2025
Related Post
Thank you for visiting our website which covers about A Process With A Calculated Positive Q . We hope the information provided has been useful to you. Feel free to contact us if you have any questions or need further assistance. See you next time and don't miss to bookmark.