An Action Potential Enters A Muscle Cell At The
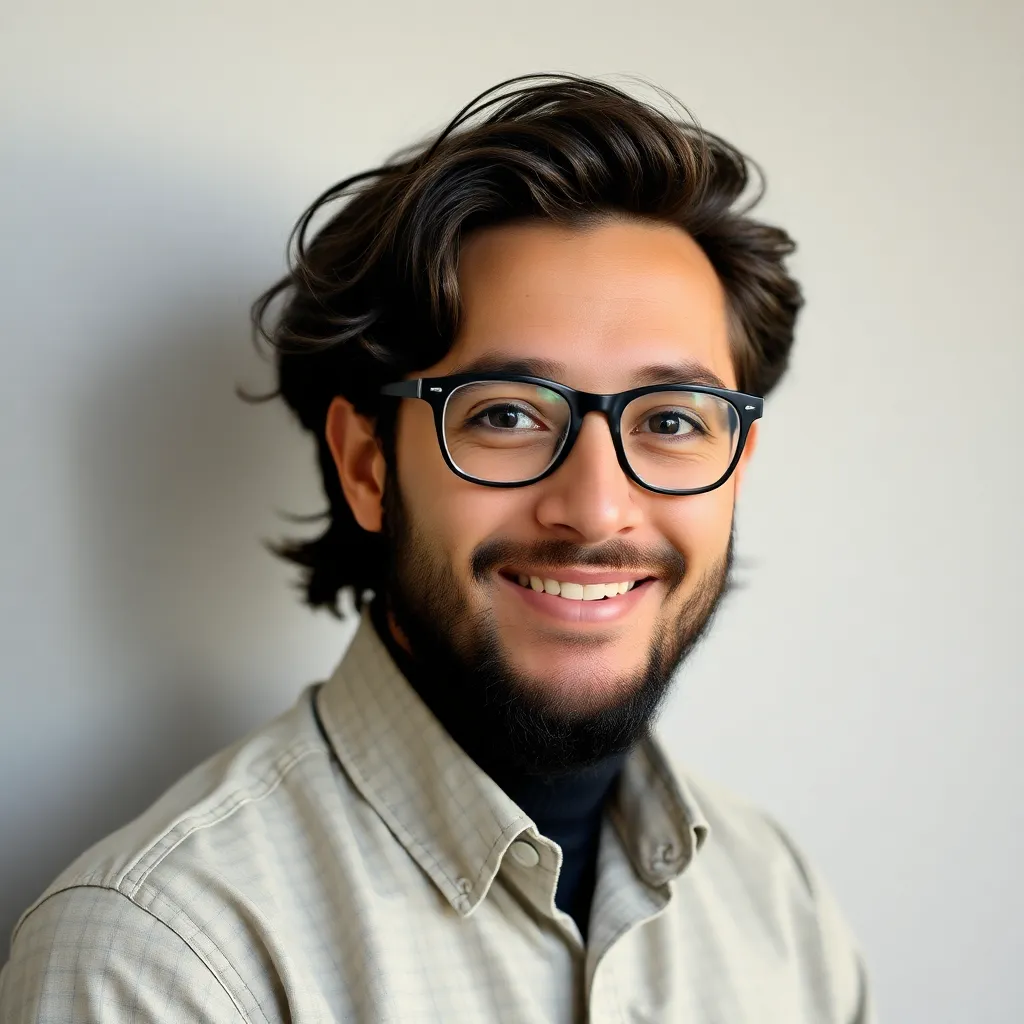
Breaking News Today
May 09, 2025 · 6 min read
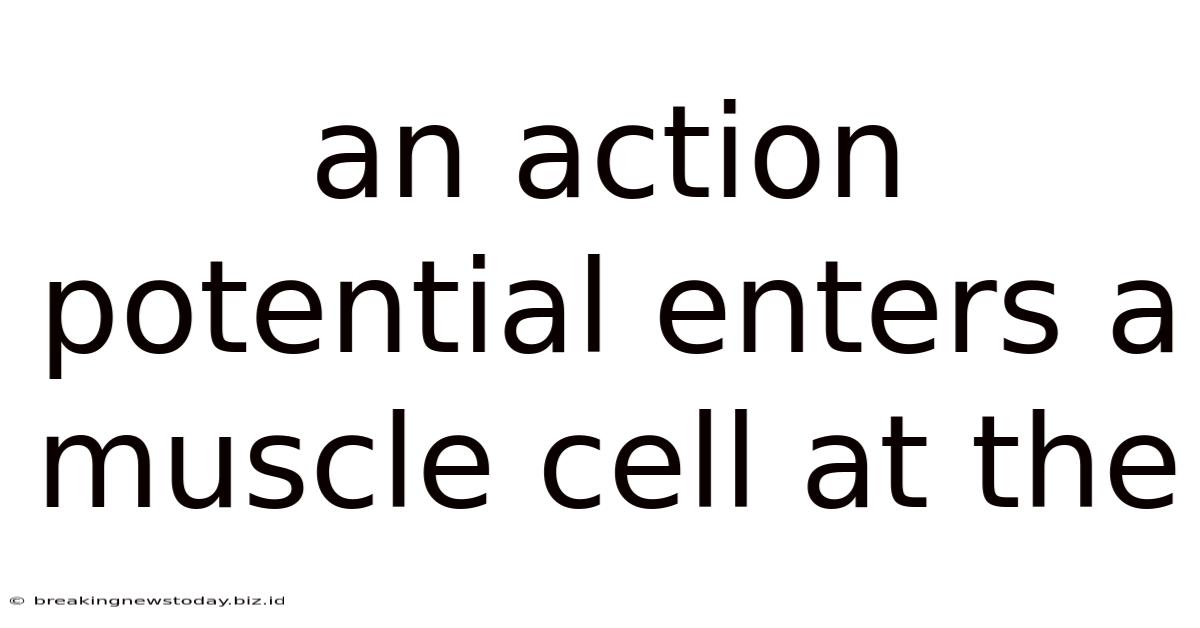
Table of Contents
An Action Potential Enters a Muscle Cell: A Deep Dive into Excitation-Contraction Coupling
The human body is a marvel of intricate biological machinery, and nowhere is this more evident than in the complex process of muscle contraction. This seemingly simple act—a flex of the bicep, a beat of the heart, the subtle twitch of an eyelid—is orchestrated by a precise sequence of events, beginning with the arrival of an action potential at the muscle cell membrane. This article will delve into the fascinating world of excitation-contraction coupling, exploring the journey of an action potential from its arrival at the neuromuscular junction to the ultimate sliding of actin and myosin filaments, leading to muscle contraction.
The Neuromuscular Junction: The First Contact
The story begins at the neuromuscular junction (NMJ), the specialized synapse between a motor neuron and a muscle fiber. This is where the nervous system communicates with the muscular system, initiating the cascade of events that culminate in muscle contraction. The process begins when an action potential, a rapid change in the electrical potential across the membrane of a nerve cell, races down the axon of a motor neuron.
Arrival and Neurotransmitter Release:
Upon reaching the axon terminal at the NMJ, the action potential triggers the opening of voltage-gated calcium channels. This influx of calcium ions into the axon terminal initiates a series of events leading to the fusion of synaptic vesicles with the presynaptic membrane. These vesicles contain acetylcholine (ACh), a neurotransmitter crucial for muscle contraction.
Acetylcholine and the Motor End Plate:
The released ACh diffuses across the synaptic cleft, the narrow gap between the axon terminal and the muscle fiber membrane, also known as the motor end plate. ACh molecules then bind to nicotinic acetylcholine receptors (nAChRs), ligand-gated ion channels located on the motor end plate.
Depolarization of the Sarcolemma:
The binding of ACh to nAChRs causes these channels to open, allowing the influx of sodium ions (Na+) into the muscle fiber and the efflux of potassium ions (K+) out of the cell. This influx of positively charged sodium ions creates a depolarization, a significant change in the membrane potential, making it less negative. This depolarization is known as the end-plate potential (EPP).
From EPP to Action Potential: Spreading the Signal
The EPP, if sufficiently strong, triggers the opening of voltage-gated sodium channels in the adjacent sarcolemma (the muscle cell membrane). This initiates a self-propagating action potential that travels along the sarcolemma, ensuring the entire muscle fiber is stimulated. This rapid spread of the action potential is essential for coordinated muscle contraction.
The Role of T-Tubules:
The action potential doesn't just stay on the surface; it dives deep into the muscle fiber's interior via specialized structures called transverse tubules (T-tubules). These invaginations of the sarcolemma extend throughout the muscle fiber, ensuring the electrical signal reaches the sarcoplasmic reticulum (SR), the intracellular calcium store.
Excitation-Contraction Coupling: Unleashing the Calcium
This is where the magic happens. The arrival of the action potential at the T-tubules triggers a crucial interaction with the SR. Specifically, the action potential activates dihydropyridine receptors (DHPRs), voltage-sensors located in the T-tubule membrane.
DHPRs and Ryanodine Receptors:
DHPRs are mechanically coupled to ryanodine receptors (RyRs), calcium release channels located on the SR membrane. The conformational change in DHPRs induced by the action potential directly activates RyRs, causing them to open. This opening allows a massive release of calcium ions (Ca2+) stored within the SR into the cytoplasm of the muscle fiber.
Calcium's Crucial Role:
The sudden increase in cytosolic calcium concentration is the key event in excitation-contraction coupling. Calcium ions bind to troponin C, a protein complex located on the thin filaments (actin) of the sarcomere, the basic contractile unit of a muscle fiber.
The Sliding Filament Theory: Contraction in Action
The binding of calcium to troponin C triggers a conformational change in the troponin-tropomyosin complex. This exposes the myosin-binding sites on the actin filaments, allowing the interaction between actin and myosin, the molecular motors responsible for muscle contraction.
Cross-Bridge Cycling:
Myosin heads, carrying ADP and inorganic phosphate (Pi), bind to the exposed myosin-binding sites on actin. This binding initiates the power stroke, a conformational change in the myosin head that pulls the actin filament towards the center of the sarcomere. ATP then binds to the myosin head, causing it to detach from actin. The hydrolysis of ATP to ADP and Pi resets the myosin head, preparing it for another cycle. This continuous cycle of attachment, power stroke, detachment, and resetting is known as cross-bridge cycling.
Sarcomere Shortening and Muscle Contraction:
The coordinated action of numerous cross-bridges along the length of the sarcomere causes the actin and myosin filaments to slide past each other, resulting in sarcomere shortening. This shortening of individual sarcomeres leads to the overall shortening of the muscle fiber and, subsequently, muscle contraction.
Relaxation: The Calcium Pump
Muscle relaxation occurs when the cytosolic calcium concentration returns to its resting level. This is achieved primarily by the sarcoplasmic/endoplasmic reticulum Ca2+-ATPase (SERCA) pump, which actively transports calcium ions back into the SR. As calcium is removed from the troponin C, the tropomyosin complex shifts back, blocking the myosin-binding sites on actin, preventing further cross-bridge cycling.
Acetylcholinesterase and the End of the Signal:
Meanwhile, at the NMJ, acetylcholinesterase (AChE), an enzyme located in the synaptic cleft, rapidly breaks down the remaining ACh. This removal of ACh from the nAChRs prevents continuous depolarization of the motor end plate, ensuring that muscle contraction is not sustained indefinitely.
Variations in Excitation-Contraction Coupling:
While the general principles of excitation-contraction coupling remain consistent across different muscle types, there are subtle variations. For instance, cardiac muscle relies on a slightly different mechanism for calcium-induced calcium release, where calcium influx through L-type calcium channels triggers further calcium release from the SR. Smooth muscle exhibits even greater diversity, with various mechanisms involved in calcium regulation and contraction.
Clinical Significance and Disorders:
Understanding excitation-contraction coupling is crucial for comprehending various neuromuscular disorders. Disruptions at any stage of this process, from neurotransmitter release to calcium handling, can lead to muscle weakness, fatigue, or paralysis. Conditions such as myasthenia gravis (affecting ACh receptors), malignant hyperthermia (affecting calcium release), and muscular dystrophies (affecting muscle fiber structure) all highlight the importance of a properly functioning excitation-contraction coupling mechanism.
Conclusion: A Symphony of Molecular Events
The journey of an action potential from its arrival at the neuromuscular junction to the generation of force in a muscle fiber is a remarkable testament to the precision and efficiency of biological processes. Excitation-contraction coupling is a carefully orchestrated dance of electrical signals, ion fluxes, and protein interactions, resulting in the coordinated movement that defines life itself. Further research into this intricate process continues to unravel the mysteries of muscle function, offering crucial insights into health, disease, and the potential for therapeutic interventions.
Latest Posts
Latest Posts
-
Advantages And Disadvantages Of Wto To Lics And Hics
May 11, 2025
-
Health Disparities Are Similarities In Health Outcomes Between Groups
May 11, 2025
-
Talk To Me Like I M Someone You Love Flashcards Pdf
May 11, 2025
-
Penalties For Your First Dui Conviction Include
May 11, 2025
-
Eliminating Sexual Harassment Is Primarily Accomplished By Applying
May 11, 2025
Related Post
Thank you for visiting our website which covers about An Action Potential Enters A Muscle Cell At The . We hope the information provided has been useful to you. Feel free to contact us if you have any questions or need further assistance. See you next time and don't miss to bookmark.