An Enzyme Is Substrate-specific Because Of The Shape Of Its
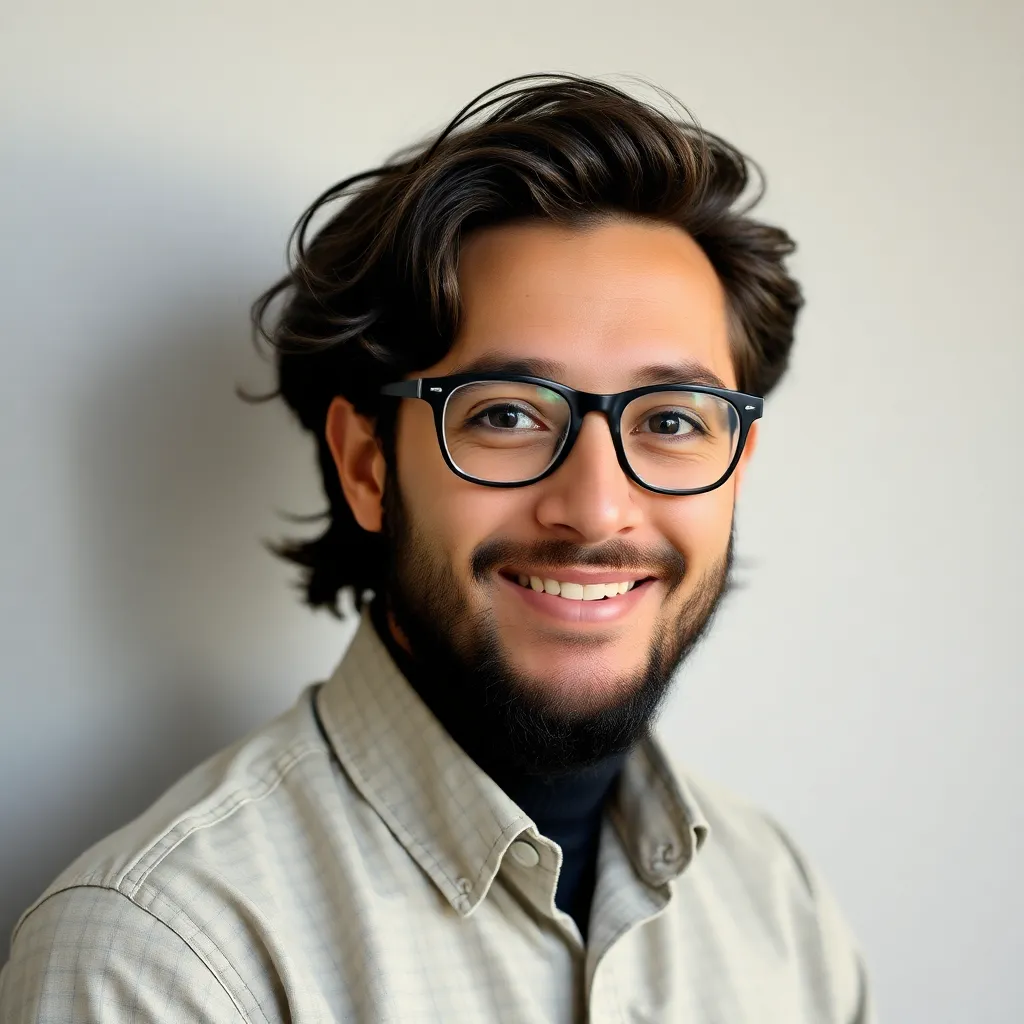
Breaking News Today
Apr 17, 2025 · 6 min read

Table of Contents
An Enzyme is Substrate-Specific Because of the Shape of its Active Site
Enzymes are biological catalysts, vital for virtually every biochemical reaction within living organisms. Their remarkable ability to accelerate reaction rates, sometimes by millions of times, hinges on their exquisite specificity. This specificity, the ability of an enzyme to selectively bind and catalyze the reaction of a specific substrate (or a small group of closely related substrates) and not others, is primarily dictated by the shape of its active site.
Understanding the Lock and Key Model and Induced Fit Model
The classical explanation for enzyme-substrate specificity is the lock and key model. This model proposes that the active site of an enzyme has a rigid, precisely defined three-dimensional shape, much like a lock. Only the substrate with a complementary shape, like a specific key, can fit into this active site, forming an enzyme-substrate complex. This interaction is highly specific, analogous to how only the correct key will open a specific lock. While simple and illustrative, the lock and key model has limitations as it doesn't account for the flexibility observed in many enzyme-substrate interactions.
A more refined and widely accepted model is the induced fit model. This model acknowledges the inherent flexibility of both the enzyme and the substrate. Upon substrate binding, the enzyme's active site undergoes a conformational change, altering its shape to better accommodate the substrate. This induced fit optimizes the interaction between the enzyme and substrate, leading to a more stable and efficient enzyme-substrate complex. This dynamic interaction enhances the specificity by allowing a degree of flexibility in substrate recognition while still maintaining high selectivity.
The Role of Non-Covalent Interactions in Substrate Binding
The specificity of enzyme-substrate binding is largely governed by a variety of weak, non-covalent interactions. These interactions, while individually weak, collectively contribute to a strong and highly specific binding affinity. These interactions include:
1. Hydrogen Bonds:
Hydrogen bonds are formed between electronegative atoms (such as oxygen or nitrogen) and a hydrogen atom covalently bonded to another electronegative atom. The precise arrangement of hydrogen bond donors and acceptors in the active site and on the substrate dictates the specificity of binding. A mismatch in hydrogen bonding patterns will result in weak or no binding.
2. Ionic Interactions (Salt Bridges):
Ionic interactions, also known as salt bridges, occur between oppositely charged groups on the enzyme and the substrate. The strength of these interactions depends on the distance and orientation of the charged groups. Specific arrangements of charged residues in the active site allow for selective attraction of substrates carrying complementary charges.
3. Hydrophobic Interactions:
Hydrophobic interactions arise from the tendency of nonpolar molecules to aggregate in an aqueous environment. Active sites often contain hydrophobic pockets that attract and bind to nonpolar regions of the substrate. The precise shape and size of these hydrophobic regions contribute significantly to substrate specificity.
4. Van der Waals Interactions:
Van der Waals forces are weak, short-range interactions that occur between atoms or molecules due to temporary fluctuations in electron distribution. While individually weak, these interactions contribute cumulatively to the overall binding affinity, particularly when many such interactions are formed between the enzyme and the substrate.
Active Site Architecture and Substrate Specificity
The active site's architecture, encompassing its three-dimensional shape, the arrangement of amino acid residues, and the presence of specific cofactors or coenzymes, are crucial determinants of substrate specificity.
1. Shape Complementarity:
The overall shape of the active site is the primary determinant of substrate specificity. Only substrates whose shape and size closely match the active site can fit effectively, forming a stable enzyme-substrate complex. Minor alterations in the substrate's shape can drastically reduce or abolish binding affinity.
2. Amino Acid Residues within the Active Site:
Specific amino acid residues within the active site directly participate in binding the substrate and catalyzing the reaction. The arrangement and properties (e.g., charge, hydrophobicity, size) of these residues dictate the types of substrates that can bind and the nature of the reaction catalyzed. For example, a catalytic triad consisting of serine, histidine, and aspartate is a common feature of serine proteases, contributing to their substrate specificity and catalytic mechanism.
3. Co-factors and Co-enzymes:
Some enzymes require cofactors (metal ions) or coenzymes (organic molecules) for activity. These molecules can directly participate in substrate binding and catalysis, further enhancing specificity. For instance, the heme group in cytochrome c oxidase plays a critical role in oxygen binding and electron transfer.
Examples of Enzyme Substrate Specificity
Numerous examples showcase the remarkable specificity of enzymes:
- Sucrase: This enzyme specifically hydrolyzes sucrose (table sugar) into glucose and fructose. It will not act on other disaccharides like lactose or maltose.
- Lactase: This enzyme specifically hydrolyzes lactose (milk sugar) into glucose and galactose. Its inability to act on sucrose or maltose illustrates substrate specificity.
- Trypsin: A serine protease, trypsin specifically cleaves peptide bonds after positively charged amino acid residues (lysine and arginine). This selective cleavage is crucial for protein digestion.
- Hexokinase: This enzyme phosphorylates hexoses like glucose, fructose, and mannose but displays varying degrees of affinity for each, reflecting its substrate specificity.
Consequences of Lack of Specificity
The high specificity of enzymes is critical for proper cellular function. Lack of specificity, or broad substrate tolerance, can lead to several detrimental consequences:
- Off-target effects: Non-specific enzymes might catalyze unwanted reactions, affecting different molecules and pathways, disrupting cellular homeostasis.
- Reduced efficiency: While some degree of promiscuity can be beneficial in certain contexts (e.g., drug development), general lack of specificity means less efficient utilization of resources.
- Toxicity: Non-specific enzymes might generate harmful byproducts, leading to cellular damage and potentially toxicity.
Studying Enzyme Specificity: Methods and Techniques
Several methods and techniques allow scientists to study enzyme specificity:
- Kinetic analysis: Measuring reaction rates at varying substrate concentrations reveals the enzyme's affinity for different substrates.
- X-ray crystallography: Determining the three-dimensional structure of enzyme-substrate complexes directly reveals the interactions contributing to substrate specificity.
- Site-directed mutagenesis: Altering specific amino acid residues within the active site allows investigation of their role in substrate binding and catalysis.
- Molecular modeling and docking: Computational approaches predict how substrates interact with enzymes, helping to understand the basis of substrate specificity.
Conclusion
Enzyme substrate specificity is a fundamental concept in biochemistry, deeply influencing metabolic pathways and cellular functions. The precise three-dimensional shape of the enzyme's active site, coupled with various non-covalent interactions, dictates which substrates can bind and undergo catalysis. The induced fit model provides a dynamic framework for understanding the flexible nature of enzyme-substrate interactions. Studying enzyme specificity is crucial for comprehending biological processes, developing new drugs and therapies, and engineering enzymes with novel properties for various applications. Understanding the intricate interplay between enzyme structure, substrate binding, and catalysis is essential for advancing our knowledge of biological systems and their regulation. Further research continues to unravel the complexities of enzyme specificity and its profound implications in biology and beyond. The ongoing development of new techniques will undoubtedly enhance our understanding of this fundamental aspect of enzymatic function. The remarkable precision and efficiency of enzymes highlight the power of natural selection in shaping biological systems to achieve exquisite control and regulation of metabolic pathways.
Latest Posts
Latest Posts
-
According To Prohibited Personnel Practices Employees Who Are Subjected
Apr 19, 2025
-
What Is The Final Electron Acceptor In The Etc
Apr 19, 2025
-
Luisa 1 Of 1 Muchas Presiones Este Ano
Apr 19, 2025
-
Calibration Of Dental X Ray Equipment Should Be Performed By A
Apr 19, 2025
-
What Is Not True About Booking Official Flights
Apr 19, 2025
Related Post
Thank you for visiting our website which covers about An Enzyme Is Substrate-specific Because Of The Shape Of Its . We hope the information provided has been useful to you. Feel free to contact us if you have any questions or need further assistance. See you next time and don't miss to bookmark.