Essentials Of Radiographic Physics And Imaging Chapter 1
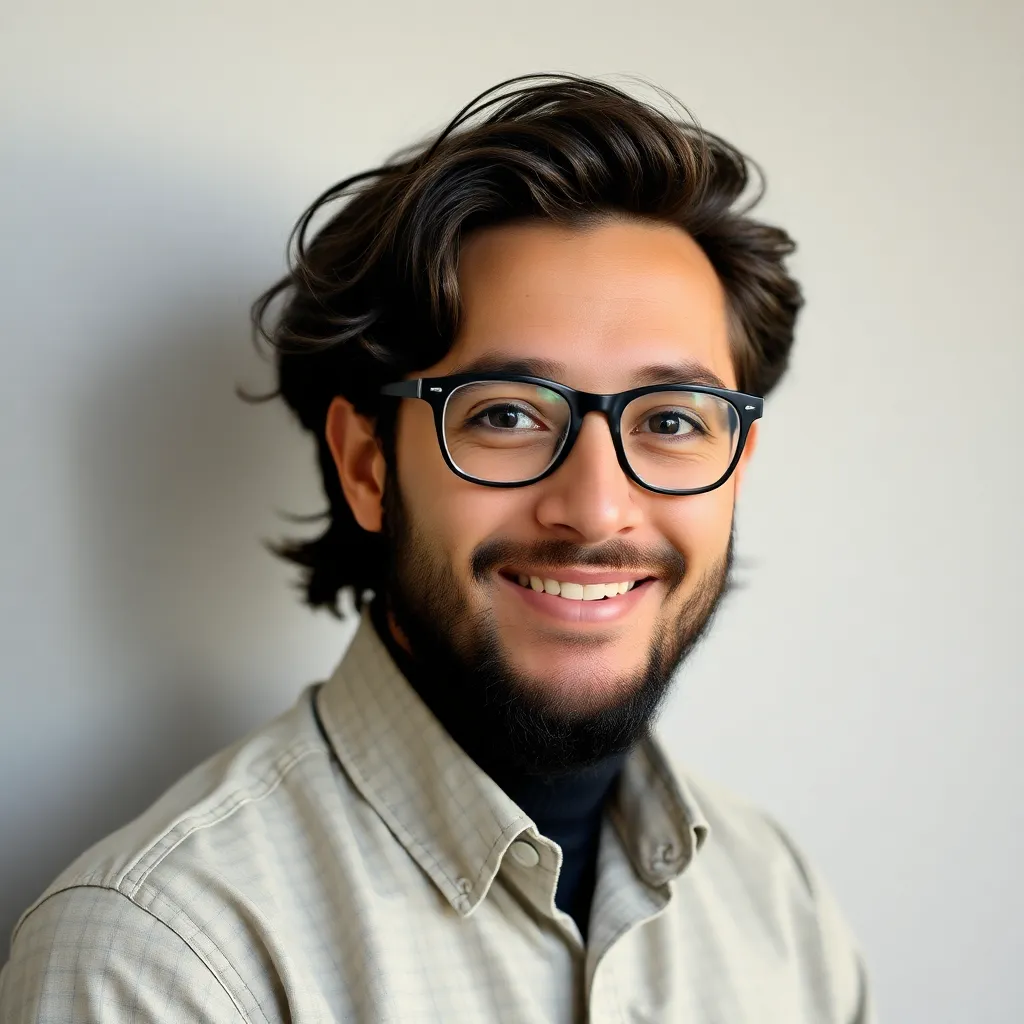
Breaking News Today
Apr 02, 2025 · 6 min read
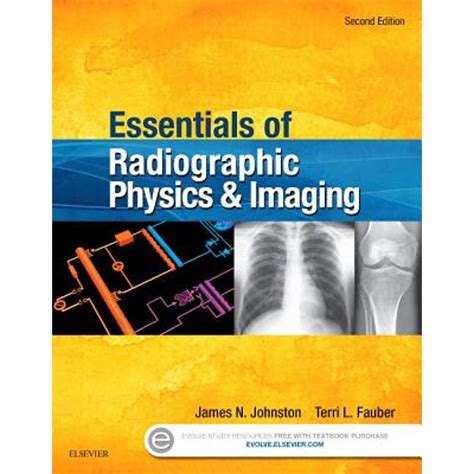
Table of Contents
Essentials of Radiographic Physics and Imaging: Chapter 1 - A Deep Dive into the Fundamentals
Welcome to the fascinating world of radiographic physics and imaging! This comprehensive guide delves into the essential principles forming the bedrock of this crucial medical field. Chapter 1 lays the groundwork, covering fundamental concepts that are vital for understanding how radiographic images are produced and interpreted. We'll explore the nature of radiation, interaction with matter, and the basic components of an x-ray imaging system.
Understanding X-Rays: The Foundation of Radiographic Imaging
At the heart of radiographic imaging lies the x-ray, a form of electromagnetic radiation. Unlike visible light, x-rays possess significantly higher energy and shorter wavelengths, enabling them to penetrate various materials. This penetrating power is precisely what allows us to visualize internal structures within the human body.
The Electromagnetic Spectrum: Where X-Rays Fit In
The electromagnetic spectrum encompasses a wide range of electromagnetic radiation, categorized by wavelength and frequency. X-rays occupy a specific region of this spectrum, nestled between gamma rays (higher energy) and ultraviolet radiation (lower energy). Understanding this placement is crucial, as it highlights the unique properties of x-rays—their ability to penetrate matter and interact with atoms in a way that allows for image formation.
X-ray Production: A Closer Look at the X-ray Tube
The production of x-rays relies on a specialized device: the x-ray tube. This sophisticated instrument consists of several key components working in concert:
- Cathode: The negatively charged electrode, typically made of tungsten, serves as the source of electrons. Heating this filament through a high current releases electrons through a process called thermionic emission.
- Anode: The positively charged electrode, also commonly made of tungsten, acts as the target for the high-speed electrons. The impact of these electrons on the anode generates x-rays. Anode designs vary, with rotating anodes common in higher-powered systems to dissipate heat more effectively.
- High Voltage Generator: This essential component accelerates the electrons emitted from the cathode towards the anode. The higher the voltage, the greater the speed of electrons and, consequently, the higher the energy of the produced x-rays.
- Collimator: Located at the output of the x-ray tube, the collimator restricts the x-ray beam to a precise size and shape, minimizing scatter radiation and improving image quality. Precise collimation is crucial for reducing patient dose and enhancing image clarity.
X-ray Interactions with Matter: The Key to Image Formation
Once generated, x-rays interact with the matter they encounter, primarily through several key mechanisms:
-
Photoelectric Absorption: This process involves the complete absorption of an x-ray photon by an inner-shell electron of an atom. The energy of the photon is transferred to the electron, ejecting it from the atom. This interaction is energy-dependent, with higher energy x-rays less likely to undergo photoelectric absorption. This is highly significant in generating image contrast, as different tissues absorb x-rays to varying degrees. Bone, with its high atomic number, absorbs significantly more x-rays than soft tissue.
-
Compton Scattering: In this interaction, an x-ray photon collides with an outer-shell electron, transferring some of its energy to the electron and scattering the photon in a new direction. This scattered radiation contributes to image degradation, known as scatter radiation, reducing image contrast and clarity. Minimizing scatter radiation is a key objective in radiographic imaging techniques.
-
Pair Production: At very high energies (above 1.02 MeV), an x-ray photon can interact with the nucleus of an atom, producing an electron-positron pair. This is less relevant in diagnostic radiography but crucial in higher-energy applications like radiotherapy.
The relative proportion of these interactions determines how much radiation is absorbed or scattered, which is fundamental to how differences in tissue density and atomic number translate into varying shades of gray on the radiographic image. Understanding these interactions is paramount to interpreting radiographic images accurately.
Image Formation and Receptors: From X-rays to Images
The x-rays that successfully penetrate the body are then captured by an image receptor, converting the invisible x-ray pattern into a visible image. Historically, film was the primary image receptor; however, modern imaging uses digital detectors significantly improving image quality and workflow.
Film-Screen Imaging: A Legacy Technology
Although largely replaced by digital methods, understanding film-screen imaging provides valuable context. X-rays interact with the phosphor crystals in intensifying screens, producing visible light. This light exposes the photographic film, creating a latent image that is then chemically processed to reveal the final radiograph. The intensifying screens amplify the x-ray signal, reducing the required radiation dose, but also introduce some image blurring.
Digital Radiography: The Modern Standard
Digital radiography uses solid-state detectors to directly capture the x-ray energy, converting it into an electronic signal. This signal is then processed by a computer, allowing for image manipulation, enhancement, and storage. Digital radiography offers significant advantages over film-screen imaging, including:
- Improved image quality: Higher spatial resolution and contrast resolution enable finer detail visualization.
- Increased efficiency: Elimination of chemical processing speeds up the workflow.
- Post-processing capabilities: Digital images can be adjusted for brightness, contrast, and other parameters.
- Electronic storage and transmission: Images can be easily stored and shared electronically.
Several types of digital detectors exist, including:
- Flat-panel detectors: These detectors consist of a large array of tiny sensors that capture x-ray energy.
- Charge-coupled devices (CCDs): These detectors use a silicon chip to convert x-rays into electrical signals.
The choice of detector influences image quality and system cost.
Image Quality: Essential Parameters
The quality of a radiographic image is determined by several key factors:
- Spatial Resolution: The ability to distinguish between two closely spaced objects. Higher spatial resolution means sharper images.
- Contrast Resolution: The ability to distinguish between different shades of gray. Higher contrast resolution means better differentiation between tissues.
- Noise: Random fluctuations in the image signal that reduce clarity. Noise is influenced by several factors including radiation dose, detector type, and image processing techniques.
- Image Magnification: The increase in image size compared to the actual object size.
- Image Distortion: Any alteration in the shape or size of the object in the image.
Radiation Safety: A Paramount Concern
Radiographic imaging involves ionizing radiation, posing potential health risks. Therefore, adhering to stringent safety protocols is paramount:
- ALARA Principle: As Low As Reasonably Achievable—this principle guides radiation protection efforts, aiming to minimize patient and staff exposure.
- Shielding: Lead aprons, gloves, and other protective barriers shield personnel from unnecessary radiation exposure.
- Collimation: Restricting the x-ray beam to the area of interest minimizes scatter radiation and reduces patient dose.
- Distance: Increasing the distance between the radiation source and the individual reduces exposure.
- Time: Minimizing exposure time reduces the overall radiation dose.
Conclusion: Building a Strong Foundation
This first chapter provides a foundational understanding of the essential principles governing radiographic physics and imaging. Grasping these fundamentals is critical for anyone working in or studying this field. From the generation of x-rays within the tube to the interaction with matter and the subsequent image formation and interpretation, each step requires a thorough understanding of the underlying physical processes. A strong grasp of these concepts forms the base upon which more complex aspects of radiographic imaging can be built. Future chapters will explore these more advanced topics in detail, expanding on the information presented here. Remember, patient safety and image quality are paramount, and understanding these basics is essential for achieving both.
Latest Posts
Latest Posts
-
What Percentage Of Your Gross Salary Does The Consumer Financial
Apr 03, 2025
-
Which Reason Best Explains Why Metals Are Shiny
Apr 03, 2025
-
Which Statement Best Describes What A Thylakoid Does During Photosynthesis
Apr 03, 2025
-
Unit 6 Consequences Of Industrialization Study Guide
Apr 03, 2025
-
Good Delivery Does Not Call Attention To Itself
Apr 03, 2025
Related Post
Thank you for visiting our website which covers about Essentials Of Radiographic Physics And Imaging Chapter 1 . We hope the information provided has been useful to you. Feel free to contact us if you have any questions or need further assistance. See you next time and don't miss to bookmark.