Identify A True Statement About Stacking Patterns.
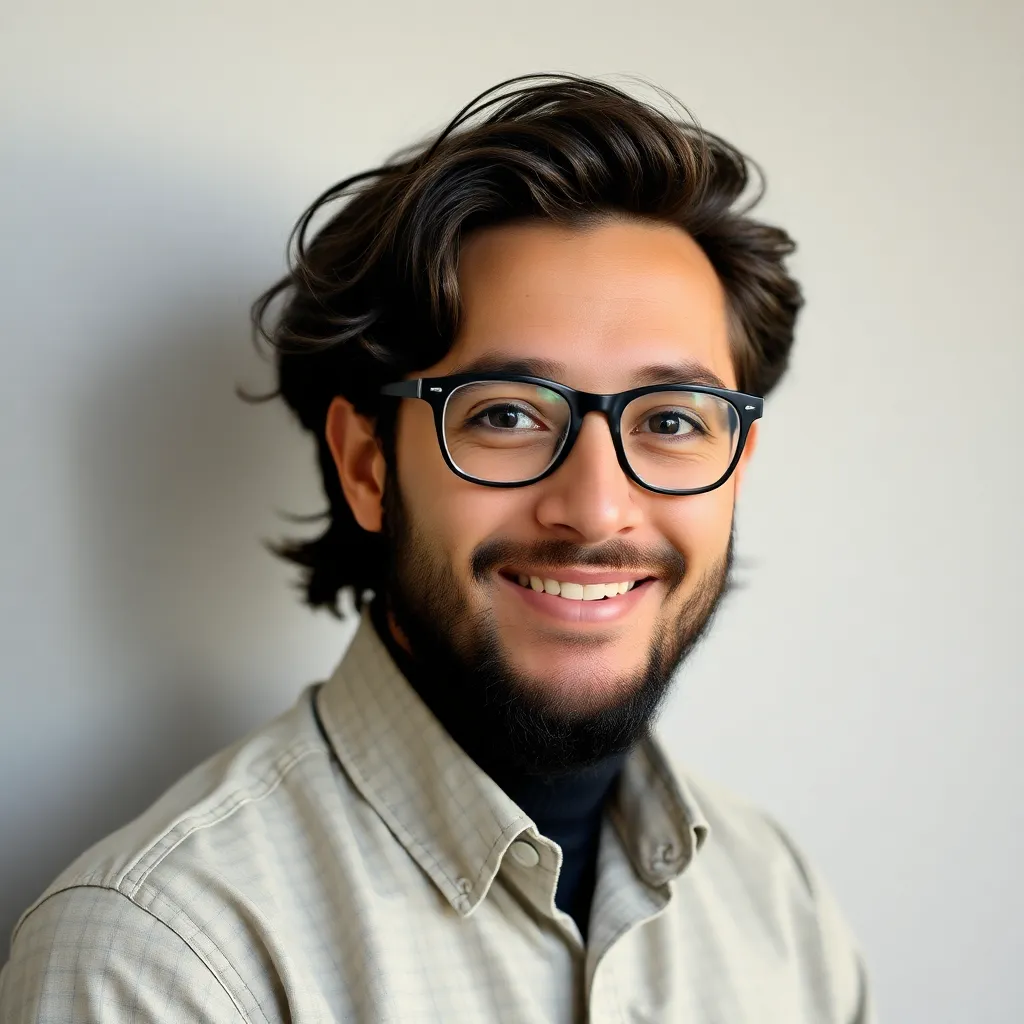
Breaking News Today
Apr 03, 2025 · 7 min read

Table of Contents
Identifying True Statements About Stacking Patterns: A Deep Dive into Material Science and Engineering
Stacking patterns, a fundamental concept in materials science and engineering, dictate the arrangement of atomic layers in crystalline structures, significantly influencing material properties. Understanding these patterns is crucial for predicting and tailoring the characteristics of various materials, from metals and alloys to ceramics and polymers. This article will delve into the intricacies of stacking patterns, identify true statements about them, and explore their implications for material behavior. We'll cover different stacking sequences, their representation using notations, and the impact on properties like ductility, strength, and electrical conductivity.
What are Stacking Patterns?
Stacking patterns describe the specific arrangement of atomic layers in close-packed crystal structures, such as those found in many metals. These structures aim to maximize atomic packing density, leading to efficient space utilization. The most common close-packed arrangements are cubic close-packed (CCP) and hexagonal close-packed (HCP). These structures are distinguished by how the subsequent atomic layers are stacked relative to the first layer.
Understanding Close-Packed Structures: A Foundation
Before delving into stacking patterns, it's important to grasp the concept of close-packed structures. In these structures, atoms are arranged in a manner that maximizes the number of nearest neighbors, resulting in high density and strong bonding. Imagine arranging spheres (representing atoms) as tightly as possible. This naturally leads to layers where each atom is surrounded by six others in a hexagonal pattern.
Representing Stacking Sequences: Notation and Interpretation
Stacking sequences are often represented using letters, commonly A, B, and C. Each letter represents a unique layer position, with each subsequent layer being shifted slightly relative to the previous one. Let's visualize this:
- Layer A: Represents the initial layer of atoms.
- Layer B: Represents a layer where atoms sit in the depressions between atoms in layer A.
- Layer C: Represents a layer where atoms sit in the remaining depressions not occupied by layer B atoms.
This simple notation system allows for concise representation of complex stacking patterns. For instance:
- ABCABCABC…: Represents a cubic close-packed (CCP) structure, also known as face-centered cubic (FCC).
- ABABABAB…: Represents a hexagonal close-packed (HCP) structure.
These notations are fundamental to understanding and comparing different stacking arrangements.
True Statements about Stacking Patterns: Fact vs. Fiction
Now, let's address some true statements about stacking patterns, separating fact from misconception:
1. Stacking patterns significantly influence material properties: This is undeniably true. The arrangement of atomic layers directly impacts mechanical properties (strength, ductility, hardness), electrical conductivity, and even magnetic properties. The different bonding arrangements and interatomic distances arising from varying stacking patterns lead to these diverse material behaviors. For instance, FCC metals are often more ductile than HCP metals due to the greater number of slip systems available for plastic deformation in FCC structures.
2. Stacking faults can arise during crystal growth: Absolutely. During the formation of crystals, imperfections can occur, resulting in deviations from the ideal stacking sequence. These deviations, called stacking faults, are planar defects within the crystal structure where the stacking sequence is interrupted (e.g., an ABCABC sequence might momentarily switch to ABCACABC). Stacking faults contribute to changes in material properties; they can increase the resistance to deformation, but also introduce sites for crack propagation, influencing the overall toughness and fatigue life.
3. The stacking pattern can be determined using X-ray diffraction: This is a cornerstone technique in materials characterization. X-ray diffraction patterns are highly sensitive to the crystal structure and stacking sequence. Specific diffraction peaks are unique to each stacking arrangement, providing direct information about the underlying atomic arrangement. By analyzing the angles and intensities of these peaks, researchers can definitively identify the stacking pattern of a given material.
4. Some materials exhibit a combination of stacking sequences: This is a crucial point that highlights the complexity and versatility of stacking patterns. It's not always a simple case of purely ABCABC or ABABAB. Some materials might exhibit mixed stacking patterns, or even a random arrangement of layers, leading to properties somewhere between purely FCC and HCP materials. This mixed stacking often arises from factors like temperature, composition, and processing techniques.
5. Stacking patterns are relevant to various material classes: While the discussion has heavily focused on metals, it's important to emphasize that stacking patterns are relevant across a broad spectrum of materials. While close-packed structures are common in metals, the principles of stacking apply to ceramics, certain polymers, and even layered compounds like graphite. In these instances, the "layers" might represent different molecular arrangements or structural units. The arrangement of these layers still has a considerable influence on overall material properties.
6. The influence of stacking patterns is not solely determined by the sequence: The impact of stacking is not merely a matter of the sequence (ABC or AB). Other factors significantly contribute. These include:
- The nature of the atoms: The size, bonding characteristics, and electronic structure of the constituent atoms drastically affect the stability of different stacking sequences.
- Temperature: At higher temperatures, the energy barrier to changing the stacking pattern can be lowered, potentially leading to a transition from one stacking arrangement to another.
- External pressure: High pressure can favor certain stacking patterns over others.
7. Stacking patterns affect the anisotropy of materials: Many materials exhibit anisotropy—a dependence of properties on direction. Stacking patterns often directly contribute to this anisotropy. Properties in the direction parallel to the stacking layers can differ significantly from properties perpendicular to the layers. This anisotropy can be exploited in material design to create materials with tailored properties in specific directions.
8. Stacking disorder affects mechanical properties differently than stacking faults: This is an important distinction. Stacking faults are localized interruptions of the stacking sequence, whereas stacking disorder refers to a more widespread and random distribution of layer arrangements. Both impact properties, but stacking disorder often results in more significant degradation of strength and ductility, compared to isolated stacking faults.
Exploring Specific Stacking Patterns and Their Impacts: Case Studies
Let's delve into specific examples of stacking patterns and their effects on material behavior:
1. Cubic Close-Packed (FCC): This structure, characterized by the ABCABC… stacking sequence, exhibits high ductility and relatively high strength due to the large number of slip systems. Materials like aluminum, copper, and nickel display this structure and are known for their malleability and formability.
2. Hexagonal Close-Packed (HCP): Represented by the ABABAB… sequence, HCP metals are generally less ductile than FCC metals, owing to the limited number of slip systems. Titanium and zinc are examples of HCP metals. Their lower ductility is often coupled with higher strength in specific directions.
3. Mixed Stacking: Many alloys exhibit mixed stacking sequences, incorporating elements of both FCC and HCP arrangements. This can lead to properties that are a blend of FCC and HCP characteristics, potentially offering a unique combination of strength, ductility, and other properties.
4. Stacking Fault Energy (SFE): The energy required to create a stacking fault is an important material parameter. Low SFE materials tend to have more extended stacking faults and are often less ductile than high SFE materials. Understanding SFE is critical in predicting and controlling material behavior.
Conclusion: The Significance of Stacking Patterns in Material Science
Stacking patterns are far more than just an abstract concept; they are a fundamental aspect of materials science that profoundly affects the properties of countless materials. Understanding these patterns, their notation, and their influence on material behavior is crucial for designing and engineering materials with specific properties for diverse applications, from aerospace and automotive to electronics and biomedicine. The ongoing research into stacking patterns and related phenomena continues to unveil new insights into the complex relationship between atomic arrangement and macroscopic properties, ultimately driving innovation in materials science and engineering.
Latest Posts
Latest Posts
-
The First Court To Hear A Case Crossword
Apr 04, 2025
-
Inaccurate Or Unhelpful Cognitions Should Be Addressed By
Apr 04, 2025
-
Hesi Case Study Management Of A Medical Unit
Apr 04, 2025
-
Which Of The Following Is True Of Public Relations
Apr 04, 2025
-
What Is The Easiest And Fastest Nail Art Medium
Apr 04, 2025
Related Post
Thank you for visiting our website which covers about Identify A True Statement About Stacking Patterns. . We hope the information provided has been useful to you. Feel free to contact us if you have any questions or need further assistance. See you next time and don't miss to bookmark.