Match Each Antibacterial Medication With Its Appropriate Mechanism Of Action.
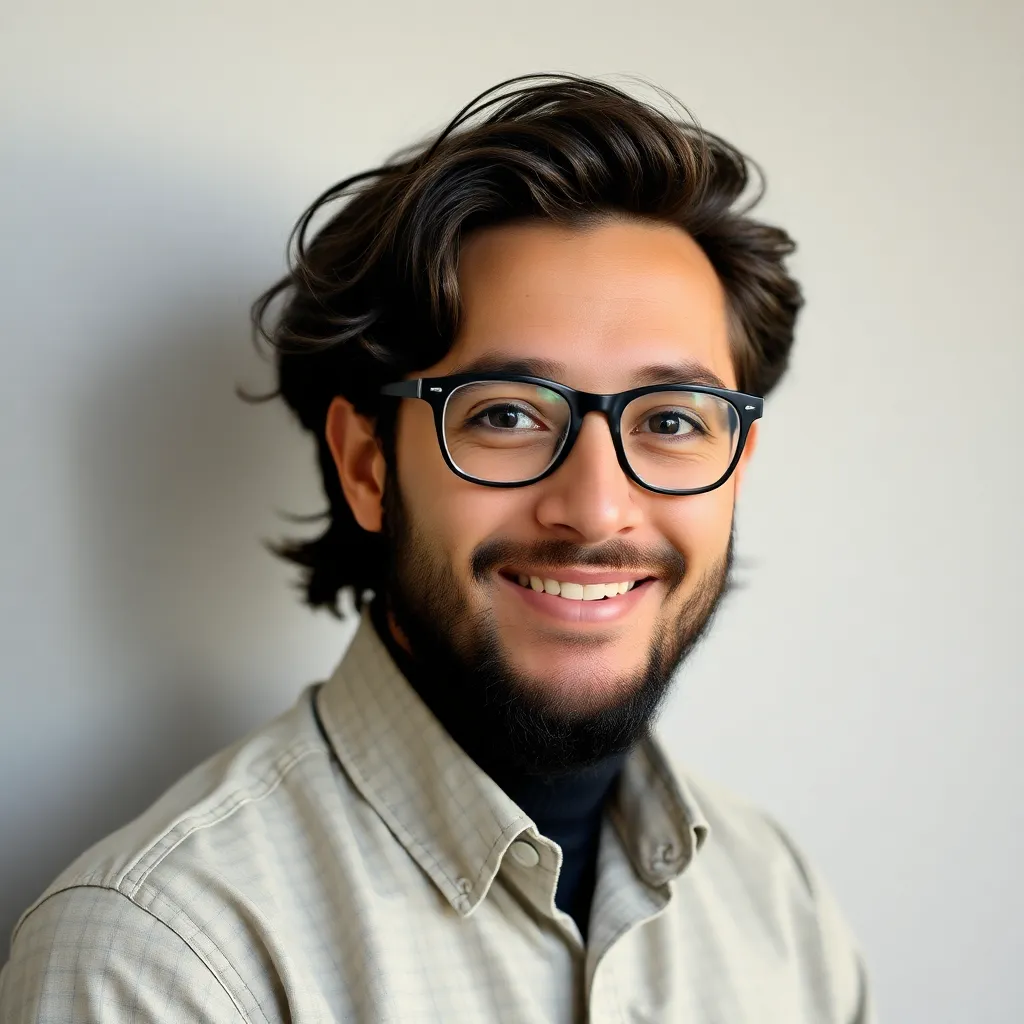
Breaking News Today
Apr 27, 2025 · 5 min read

Table of Contents
Matching Antibacterial Medications with Their Mechanisms of Action: A Comprehensive Guide
Antibacterial medications, also known as antibiotics, are crucial in combating bacterial infections. Understanding their mechanisms of action is vital for effective treatment and preventing the development of antibiotic resistance. This comprehensive guide will match various antibacterial medications with their corresponding mechanisms of action, providing a detailed overview of how these drugs work at a molecular level. We'll explore the diverse ways antibiotics target bacterial cells, leading to their demise.
Key Mechanisms of Antibacterial Action
Before delving into specific drugs, let's review the principal ways antibiotics work:
1. Inhibition of Cell Wall Synthesis:
This is a cornerstone of antibacterial action. Bacterial cell walls are crucial for maintaining cell shape and integrity. Interfering with their construction leads to cell lysis (rupture) and death. Key classes of antibiotics targeting this process include:
-
Beta-lactams (Penicillins, Cephalosporins, Carbapenems, Monobactams): These drugs inhibit penicillin-binding proteins (PBPs), enzymes essential for peptidoglycan synthesis, the main component of bacterial cell walls. Different beta-lactams have varying affinities for different PBPs, explaining their spectrum of activity.
- Penicillins (e.g., Amoxicillin, Methicillin): Effective against Gram-positive bacteria, with some penicillins exhibiting activity against Gram-negative bacteria. Their efficacy can be hampered by beta-lactamases, enzymes produced by some bacteria that break down beta-lactam rings.
- Cephalosporins (e.g., Cefazolin, Ceftriaxone): A broad spectrum of activity, including both Gram-positive and Gram-negative bacteria. They are often preferred over penicillins in cases of penicillin allergy or beta-lactamase production. Multiple generations of cephalosporins exist, with each generation showing increased resistance to beta-lactamases and broader activity against Gram-negative bacteria.
- Carbapenems (e.g., Imipenem, Meropenem): Known for their broad-spectrum activity and resistance to many beta-lactamases, they are reserved for serious infections caused by multi-drug resistant bacteria.
- Monobactams (e.g., Aztreonam): Primarily active against Gram-negative aerobic bacteria. They are often used in patients with penicillin allergies.
-
Glycopeptides (e.g., Vancomycin, Teicoplanin): These bind to the D-alanyl-D-alanine terminus of peptidoglycan precursors, preventing their incorporation into the cell wall. They are particularly effective against Gram-positive bacteria, including methicillin-resistant Staphylococcus aureus (MRSA). Vancomycin is a crucial antibiotic for treating severe infections caused by resistant Gram-positive bacteria.
-
Lipopeptides (e.g., Daptomycin): These drugs insert into the bacterial cytoplasmic membrane, causing membrane depolarization and cell death. They are primarily effective against Gram-positive bacteria. Daptomycin is another important option for treating infections caused by multi-drug-resistant Gram-positive bacteria.
-
Bacitracin: This topical antibiotic interferes with bactoprenol, a lipid carrier molecule essential for transporting peptidoglycan precursors across the cytoplasmic membrane. It's primarily used topically due to its nephrotoxicity.
2. Inhibition of Protein Synthesis:
Bacterial ribosomes are the sites of protein synthesis. Many antibiotics target these structures, disrupting protein production and leading to bacterial death. The major classes include:
-
Aminoglycosides (e.g., Gentamicin, Tobramycin, Amikacin): These drugs bind to the 30S ribosomal subunit, causing misreading of mRNA and inhibiting protein synthesis. They are primarily effective against aerobic Gram-negative bacteria and some Gram-positive bacteria. Their use is often limited by potential nephrotoxicity and ototoxicity (damage to the hearing and balance organs).
-
Tetracyclines (e.g., Tetracycline, Doxycycline, Minocycline): These antibiotics bind to the 30S ribosomal subunit, blocking the attachment of tRNA to the mRNA-ribosome complex, thereby inhibiting protein synthesis. They exhibit broad-spectrum activity, including against Gram-positive and Gram-negative bacteria, as well as some atypical bacteria and intracellular pathogens.
-
Macrolides (e.g., Erythromycin, Azithromycin, Clarithromycin): These bind to the 50S ribosomal subunit, inhibiting translocation, the movement of the ribosome along the mRNA during protein synthesis. They are effective against many Gram-positive bacteria and some Gram-negative bacteria, and are often used as alternatives to penicillins in penicillin-allergic patients.
-
Chloramphenicol: This broad-spectrum antibiotic binds to the 50S ribosomal subunit, inhibiting peptidyl transferase activity, a crucial step in peptide bond formation during protein synthesis. Its use is limited due to its potential for serious side effects, including aplastic anemia (a serious blood disorder).
-
Lincosamides (e.g., Clindamycin, Lincomycin): These antibiotics bind to the 50S ribosomal subunit, inhibiting peptide bond formation. They are effective against a range of Gram-positive and some Gram-negative anaerobic bacteria.
-
Oxazolidinones (e.g., Linezolid): These bind to the 50S ribosomal subunit, preventing the formation of the initiation complex for protein synthesis. They are effective against Gram-positive bacteria, including MRSA and vancomycin-resistant enterococci (VRE).
3. Inhibition of Nucleic Acid Synthesis:
Some antibiotics target the processes involved in bacterial DNA replication and RNA transcription, leading to impaired bacterial growth and death.
-
Quinolones (e.g., Ciprofloxacin, Levofloxacin, Moxifloxacin): These drugs inhibit DNA gyrase and topoisomerase IV, enzymes essential for DNA replication and supercoiling. They have broad-spectrum activity against both Gram-positive and Gram-negative bacteria.
-
Rifampin: This antibiotic inhibits bacterial DNA-dependent RNA polymerase, preventing the transcription of RNA from DNA. It is effective against a wide range of bacteria, including Mycobacterium tuberculosis. It's often used in combination with other drugs for the treatment of tuberculosis.
-
Metronidazole: This drug interferes with DNA synthesis in anaerobic bacteria and protozoa, leading to DNA damage and cell death. It's commonly used to treat infections caused by anaerobic bacteria and certain parasitic infections.
4. Inhibition of Metabolic Pathways:
Some antibiotics target specific metabolic pathways essential for bacterial survival.
-
Sulfonamides (e.g., Sulfamethoxazole): These drugs inhibit dihydropteroate synthase, an enzyme involved in folic acid synthesis. Bacteria require folic acid for DNA and RNA synthesis. They are often used in combination with trimethoprim (see below).
-
Trimethoprim: This antibiotic inhibits dihydrofolate reductase, another enzyme involved in folic acid synthesis. The combination of sulfamethoxazole and trimethoprim (co-trimoxazole) is synergistic, meaning that their combined effect is greater than the sum of their individual effects.
Conclusion: A Dynamic Landscape
The fight against bacterial infections is a continuous battle, with bacteria constantly evolving resistance mechanisms. Understanding the specific mechanisms of action of various antibacterial medications is crucial for rational antibiotic prescribing, optimizing treatment outcomes, and mitigating the global threat of antibiotic resistance. This guide serves as a starting point for healthcare professionals and students alike to navigate the complex world of antibacterial medications. Further research and exploration into individual drugs within each class will provide a deeper, more nuanced understanding of their clinical applications and limitations. Remember always to consult with a healthcare professional before starting or stopping any medication.
Latest Posts
Latest Posts
-
Nims Components Are Adaptable To Planned Events
Apr 27, 2025
-
Draw Trans 1 Ethyl 2 Methylcyclohexane In Its Lowest Energy Conformation
Apr 27, 2025
-
A Rational Person Does Not Act Unless
Apr 27, 2025
-
Correctly Label The Following Anatomical Features Of The Stomach
Apr 27, 2025
-
What Should Coach Kelly Do At This Point
Apr 27, 2025
Related Post
Thank you for visiting our website which covers about Match Each Antibacterial Medication With Its Appropriate Mechanism Of Action. . We hope the information provided has been useful to you. Feel free to contact us if you have any questions or need further assistance. See you next time and don't miss to bookmark.