Produces A Usable Form Of Energy For The Cell
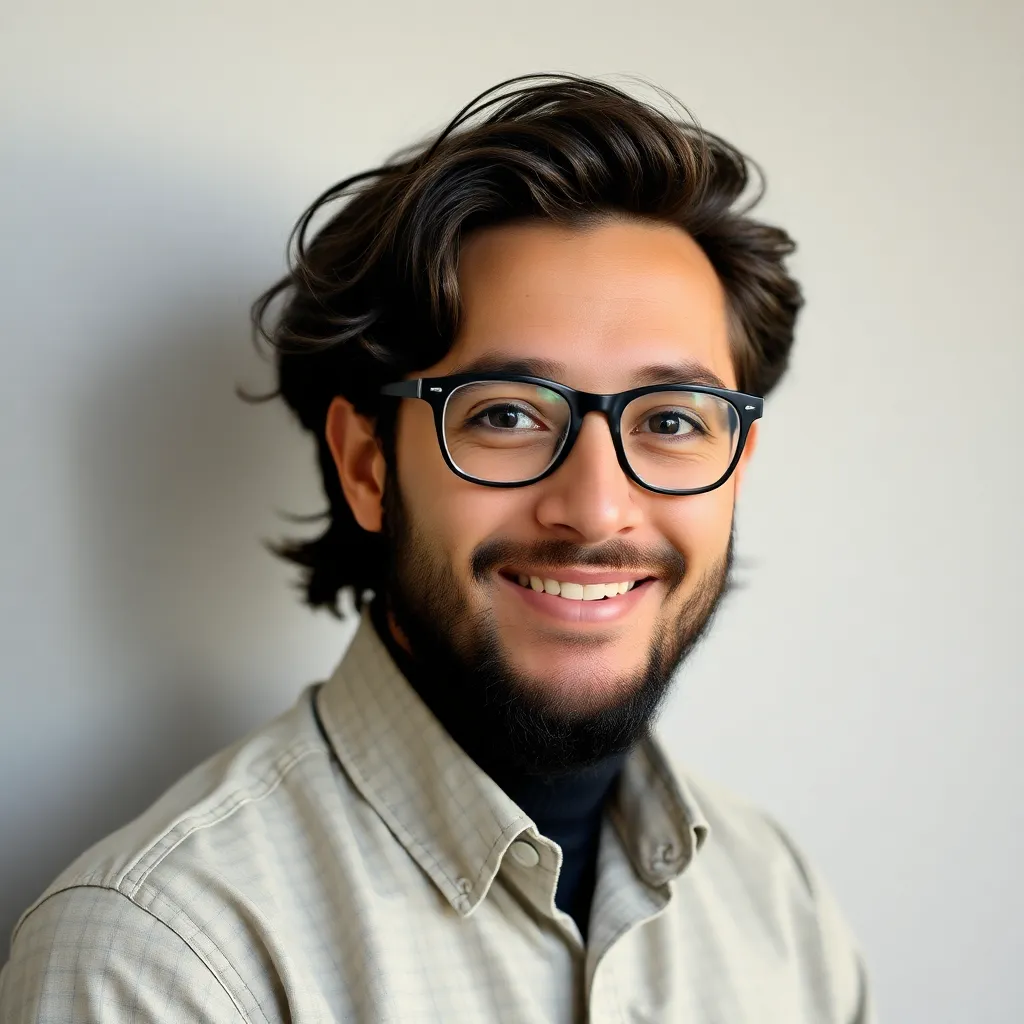
Breaking News Today
May 10, 2025 · 7 min read
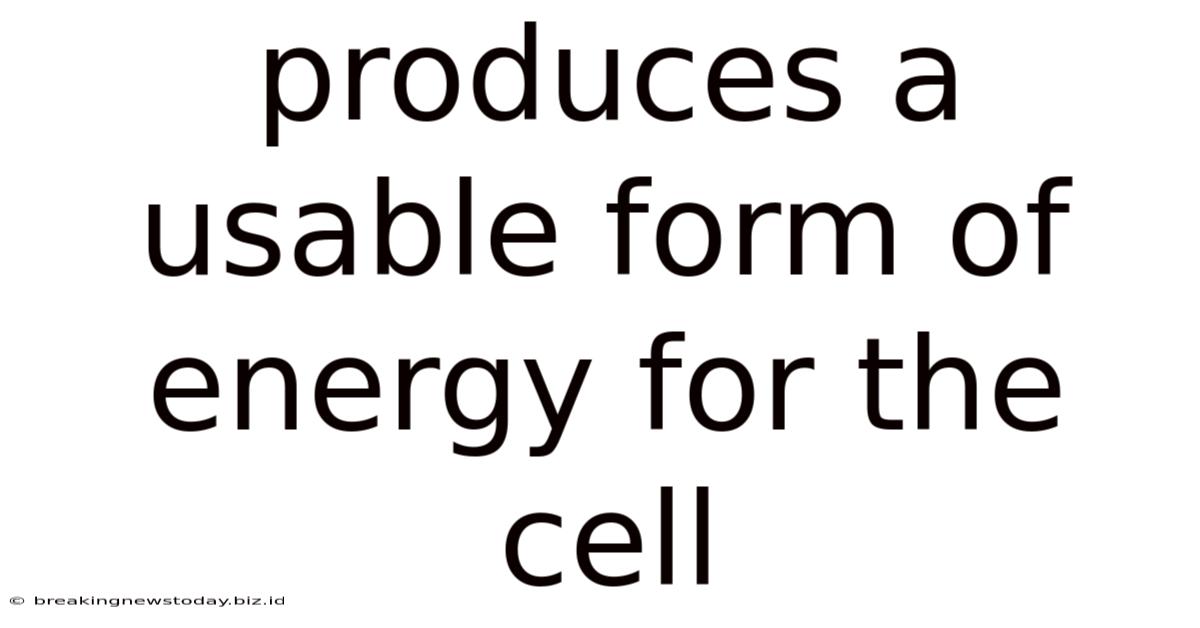
Table of Contents
ATP: The Universal Energy Currency of the Cell
Cells, the fundamental building blocks of life, are incredibly complex and dynamic entities. To perform their myriad functions – from synthesizing proteins and replicating DNA to transporting molecules and contracting muscles – they require a constant supply of energy. This energy is not stored in a raw, usable form, but rather in a specialized molecule known as adenosine triphosphate (ATP). ATP is often described as the "energy currency" of the cell because it's the primary means by which energy is transferred from energy-releasing processes to energy-requiring processes. Understanding how ATP is produced and utilized is crucial to understanding the very essence of life itself.
The Structure and Function of ATP
Before diving into the intricate pathways of ATP production, let's examine the molecule itself. ATP is a nucleotide composed of three key components:
- Adenine: A nitrogenous base, a crucial component of DNA and RNA.
- Ribose: A five-carbon sugar, forming the backbone of the molecule.
- Three phosphate groups: These are the key to ATP's energy-carrying capacity. The bonds connecting these phosphate groups are high-energy phosphate bonds, meaning they release a significant amount of energy when broken.
This unique structure allows ATP to act as an intermediary, effectively coupling energy-releasing reactions (catabolism) to energy-requiring reactions (anabolism). When a phosphate group is cleaved from ATP, forming adenosine diphosphate (ADP) and inorganic phosphate (Pi), energy is released. This energy can then be used to drive various cellular processes. Conversely, ADP can be re-phosphorylated to regenerate ATP, completing the energy cycle.
The Role of High-Energy Phosphate Bonds
The high-energy phosphate bonds in ATP are not literally "high energy" in a thermodynamic sense, but rather represent a relatively unstable configuration. The negative charges of the phosphate groups repel each other, creating a state of high potential energy. Hydrolyzing these bonds (breaking them with water) releases this potential energy, making it available for cellular work.
Major Pathways of ATP Production: Cellular Respiration
Cells primarily produce ATP through a series of metabolic pathways collectively known as cellular respiration. This process involves the breakdown of organic molecules, such as glucose, to generate ATP. Cellular respiration can be broadly categorized into four stages:
1. Glycolysis: The First Step in Energy Extraction
Glycolysis, meaning "sugar splitting," is an anaerobic process (occurs without oxygen) that takes place in the cytoplasm. It involves the breakdown of a single glucose molecule into two molecules of pyruvate. While glycolysis itself produces only a small net yield of ATP (2 molecules), it lays the foundation for subsequent, more energy-efficient stages of respiration. Furthermore, it generates NADH, a crucial electron carrier molecule, which plays a vital role in subsequent ATP production.
2. Pyruvate Oxidation: Preparing for the Citric Acid Cycle
Before pyruvate can enter the next stage, it must be converted to acetyl-CoA. This process occurs in the mitochondrial matrix (the inner compartment of the mitochondria) and involves the removal of a carbon dioxide molecule and the addition of coenzyme A. This step also generates more NADH.
3. The Citric Acid Cycle (Krebs Cycle): A Central Metabolic Hub
The citric acid cycle, a series of enzymatic reactions, takes place in the mitochondrial matrix. Acetyl-CoA enters the cycle and undergoes a series of oxidation and reduction reactions. This cycle generates a small amount of ATP directly (2 molecules) but, more importantly, produces significant amounts of NADH and FADH2, another electron carrier molecule. These electron carriers are crucial for the next stage – oxidative phosphorylation.
4. Oxidative Phosphorylation: The Major ATP Producer
Oxidative phosphorylation is the final and most significant stage of cellular respiration. It occurs in the inner mitochondrial membrane and involves two main processes:
-
Electron Transport Chain (ETC): Electrons carried by NADH and FADH2 are passed along a series of protein complexes embedded in the inner mitochondrial membrane. As electrons move down the chain, energy is released and used to pump protons (H+) from the mitochondrial matrix into the intermembrane space, creating a proton gradient.
-
Chemiosmosis: The proton gradient generated by the ETC represents stored potential energy. This gradient drives protons back into the matrix through ATP synthase, a protein complex that acts as a molecular turbine. The flow of protons through ATP synthase powers the synthesis of ATP from ADP and Pi. This process is called chemiosmosis and is responsible for the majority of ATP produced during cellular respiration. The exact number of ATP molecules produced varies slightly depending on the shuttle system used to transport electrons from NADH in the cytoplasm to the mitochondria.
Alternative Pathways of ATP Production: Fermentation
When oxygen is scarce, cells can resort to alternative pathways of ATP production, such as fermentation. Fermentation is an anaerobic process that generates ATP through glycolysis only. Since the electron transport chain is not utilized, the yield of ATP is significantly lower than in cellular respiration.
There are several types of fermentation, including lactic acid fermentation (producing lactic acid as a byproduct) and alcoholic fermentation (producing ethanol and carbon dioxide). While these processes produce less ATP, they allow cells to survive in oxygen-deprived environments.
Regulation of ATP Production: Maintaining Energy Balance
Cells must carefully regulate ATP production to meet their energy demands while avoiding wasteful overproduction. This regulation occurs at multiple levels, including:
-
Substrate Availability: The availability of glucose and other fuels directly influences the rate of glycolysis and subsequent stages of respiration.
-
Enzyme Activity: The activity of key enzymes involved in the metabolic pathways is regulated by allosteric mechanisms and feedback inhibition. For example, high levels of ATP can inhibit enzymes involved in glycolysis, slowing down ATP production.
-
Hormonal Control: Hormones like insulin and glucagon play crucial roles in regulating blood glucose levels and, consequently, the rate of ATP production.
-
Oxygen Availability: Oxygen is the final electron acceptor in the electron transport chain. Its availability directly affects the rate of oxidative phosphorylation and overall ATP production.
ATP Utilization: Powering Cellular Processes
The ATP generated through cellular respiration fuels a vast array of cellular processes, including:
-
Muscle Contraction: ATP powers the interaction between actin and myosin filaments, leading to muscle contraction.
-
Active Transport: ATP drives the movement of molecules against their concentration gradients, such as the sodium-potassium pump.
-
Protein Synthesis: ATP provides energy for the synthesis of proteins from amino acids.
-
DNA Replication and Repair: ATP is essential for DNA replication, repair, and transcription.
-
Signal Transduction: ATP is involved in signal transduction pathways, enabling cells to respond to their environment.
-
Nerve Impulse Transmission: ATP is crucial for the transmission of nerve impulses.
-
Biosynthesis: ATP provides energy for the synthesis of many essential molecules, including carbohydrates, lipids, and nucleic acids.
ATP and Disease: Dysfunctional Energy Production
Disruptions in ATP production can have severe consequences, leading to various diseases and disorders. Examples include:
-
Mitochondrial Diseases: These are a group of genetic disorders affecting the mitochondria's ability to produce ATP. Symptoms can vary widely, depending on the specific gene affected.
-
Metabolic Disorders: Conditions such as diabetes mellitus and glycogen storage diseases can affect glucose metabolism and ATP production.
-
Cancer: Cancer cells often exhibit altered metabolism, with increased glucose uptake and ATP production to support their rapid growth and proliferation.
-
Neurodegenerative Diseases: Disruptions in ATP production are implicated in neurodegenerative diseases such as Alzheimer's and Parkinson's disease.
Conclusion: The Vital Role of ATP in Life
Adenosine triphosphate (ATP) stands as a cornerstone of cellular function, acting as the universal energy currency that powers the countless processes that sustain life. Its production through intricate metabolic pathways, primarily cellular respiration, provides the necessary energy for cell growth, maintenance, and reproduction. Understanding ATP's structure, generation, and utilization is fundamental to appreciating the complexity and elegance of biological systems, and disruptions in its production can have significant implications for human health. Further research into the intricacies of ATP metabolism continues to unveil new insights into cellular processes and holds the potential for developing novel therapeutic strategies for a wide range of diseases.
Latest Posts
Latest Posts
-
It Professionals Have Strict Legal And Ethical Standards Because Of
May 10, 2025
-
Match The Term With Its Definition Gray Matter
May 10, 2025
-
Which Of The Following Statements About Enzymes Is False
May 10, 2025
-
How Does Equianos Youth Affect His Treatment During The Voyage
May 10, 2025
-
Why Is Mobile Banking Considered Riskier Than Online Banking
May 10, 2025
Related Post
Thank you for visiting our website which covers about Produces A Usable Form Of Energy For The Cell . We hope the information provided has been useful to you. Feel free to contact us if you have any questions or need further assistance. See you next time and don't miss to bookmark.