Protein Polymers Are Made Up Of Blank Monomers
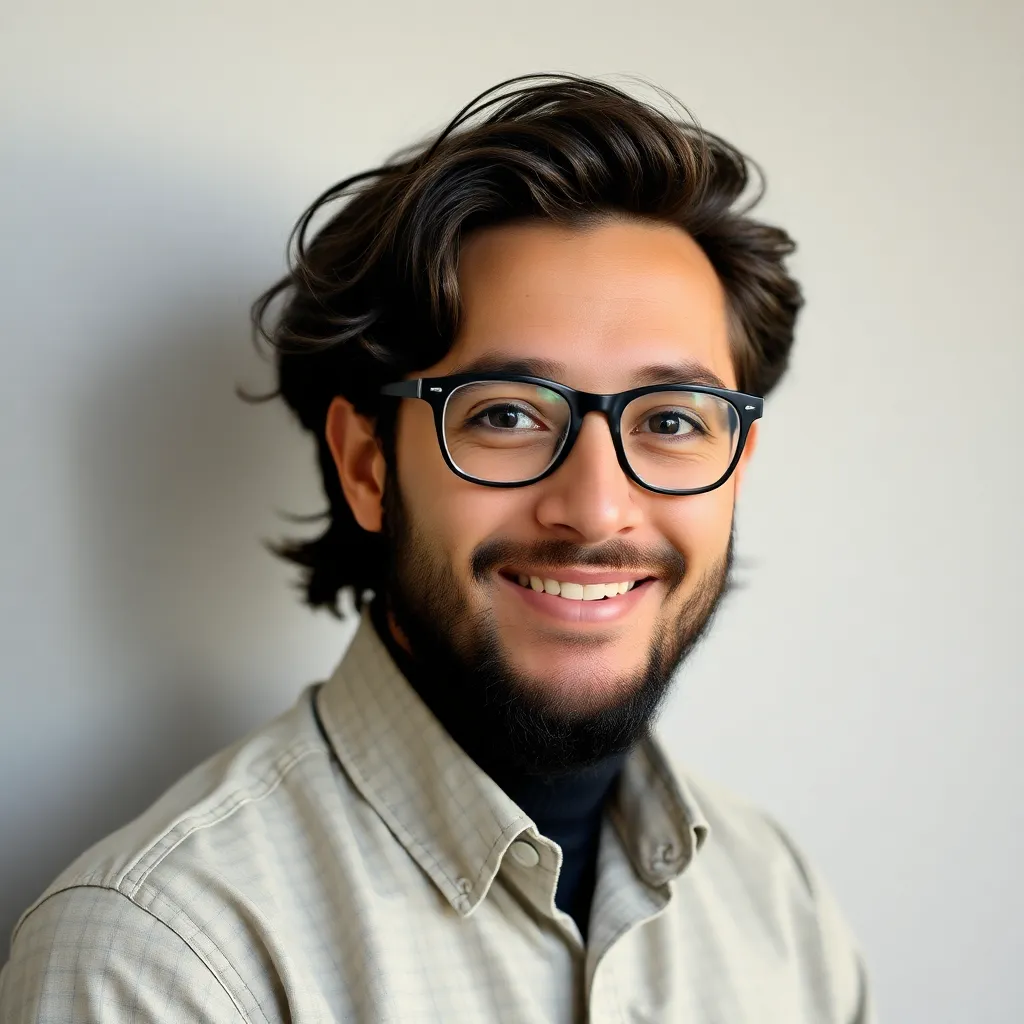
Breaking News Today
Apr 04, 2025 · 6 min read

Table of Contents
Protein Polymers: Chains of Amino Acid Monomers
Proteins are the workhorses of the cell, carrying out a vast array of functions essential for life. From catalyzing biochemical reactions (enzymes) to providing structural support (collagen), proteins' diverse roles stem from their incredibly complex structures. But at the heart of this complexity lies a simple truth: protein polymers are made up of amino acid monomers. Understanding this fundamental building block is crucial to grasping the intricacies of protein structure and function.
The Amino Acid Monomer: The Building Block of Proteins
Amino acids are organic molecules containing a central carbon atom (the alpha carbon) bonded to four groups:
- An amino group (-NH2): This group is basic, meaning it can accept a proton (H+).
- A carboxyl group (-COOH): This group is acidic, meaning it can donate a proton (H+).
- A hydrogen atom (-H): A simple hydrogen atom.
- A variable side chain (R-group): This is the unique part of each amino acid, determining its chemical properties and influencing the overall protein structure.
The R-group can be anything from a simple hydrogen atom (as in glycine) to a complex aromatic ring structure (as in tryptophan). This diversity in R-groups leads to the existence of 20 standard amino acids, each with distinct chemical characteristics:
- Nonpolar, aliphatic: Glycine (Gly, G), Alanine (Ala, A), Valine (Val, V), Leucine (Leu, L), Isoleucine (Ile, I), Methionine (Met, M)
- Aromatic: Phenylalanine (Phe, F), Tyrosine (Tyr, Y), Tryptophan (Trp, W)
- Polar, uncharged: Serine (Ser, S), Threonine (Thr, T), Cysteine (Cys, C), Asparagine (Asn, N), Glutamine (Gln, Q)
- Positively charged: Lysine (Lys, K), Arginine (Arg, R), Histidine (His, H)
- Negatively charged: Aspartate (Asp, D), Glutamate (Glu, E)
These diverse amino acids provide the raw material for the astonishing variety of proteins found in nature.
Peptide Bonds: Linking Amino Acids
Amino acids link together to form polypeptide chains through a process called dehydration synthesis. In this reaction, the carboxyl group of one amino acid reacts with the amino group of another amino acid, releasing a water molecule and forming a peptide bond (also known as an amide bond). This bond is a strong covalent bond that holds the amino acids together in the polypeptide chain. The sequence of amino acids in a polypeptide chain is known as the primary structure of the protein.
From Monomers to Polymers: Levels of Protein Structure
The primary structure – the linear sequence of amino acids – dictates the higher-order structures of a protein. These higher-order structures are crucial for the protein's function:
1. Primary Structure: The Amino Acid Sequence
As mentioned above, the primary structure is simply the sequence of amino acids linked by peptide bonds. This sequence is determined by the genetic code, with DNA specifying the order in which amino acids are added to the growing polypeptide chain during protein synthesis. Even a single amino acid substitution can have dramatic consequences, as seen in sickle cell anemia, where a single amino acid change in hemoglobin alters its function and leads to a debilitating disease. The primary structure is fundamental, as it determines all subsequent levels of protein organization.
2. Secondary Structure: Local Folding Patterns
The primary structure doesn't exist as a flat, extended chain. Instead, it folds into local structures stabilized by hydrogen bonds between the amino and carboxyl groups of the peptide backbone. The two most common secondary structures are:
- Alpha-helices: These are right-handed coiled structures stabilized by hydrogen bonds between every fourth amino acid. The R-groups project outwards from the helix.
- Beta-sheets: These are planar structures formed by hydrogen bonds between adjacent polypeptide chains (or segments of the same chain). The R-groups alternate above and below the plane of the sheet. Beta-sheets can be parallel (chains running in the same direction) or antiparallel (chains running in opposite directions).
These secondary structures provide regions of local order and stability within the protein.
3. Tertiary Structure: The 3D Conformation
The tertiary structure refers to the overall three-dimensional arrangement of a polypeptide chain, including its secondary structural elements. This structure is stabilized by a variety of interactions, including:
- Disulfide bonds: Covalent bonds between cysteine residues. These strong bonds help to stabilize the protein's folded structure.
- Hydrophobic interactions: Nonpolar R-groups cluster together in the protein's interior, away from the aqueous environment.
- Hydrogen bonds: These form between various polar R-groups and the peptide backbone.
- Ionic interactions (salt bridges): Electrostatic interactions between positively and negatively charged R-groups.
The tertiary structure is crucial for protein function, determining the shape of the active site in enzymes, the binding sites for ligands, and the overall interactions with other molecules.
4. Quaternary Structure: Multi-Subunit Proteins
Some proteins consist of multiple polypeptide chains (subunits) that assemble to form a functional protein complex. This arrangement is called the quaternary structure. The subunits are held together by the same types of interactions that stabilize tertiary structure – disulfide bonds, hydrophobic interactions, hydrogen bonds, and ionic interactions. Examples of proteins with quaternary structure include hemoglobin (four subunits) and many enzymes.
The quaternary structure allows for cooperative interactions between subunits, enhancing the protein's function or regulatory properties.
The Importance of Protein Structure and Function
The precise three-dimensional structure of a protein is intimately linked to its function. Even small changes in the amino acid sequence can disrupt the protein's folding, leading to loss of function or the formation of misfolded proteins associated with diseases like Alzheimer's and Parkinson's. The intricate interplay of forces that govern protein folding remains a fascinating area of research. Understanding the relationship between protein structure and function is crucial for developing new drugs, therapies, and biotechnological applications.
Protein Synthesis: From Gene to Protein
The process of protein synthesis involves translating the genetic information encoded in DNA into a polypeptide chain. This involves several steps:
- Transcription: The DNA sequence of a gene is transcribed into messenger RNA (mRNA).
- Translation: The mRNA sequence is translated into a polypeptide chain by ribosomes. Each three-nucleotide codon on the mRNA specifies a particular amino acid. Transfer RNA (tRNA) molecules bring the appropriate amino acids to the ribosome, where they are added to the growing polypeptide chain.
- Folding and Post-Translational Modification: After synthesis, the polypeptide chain folds into its three-dimensional structure. This process can involve chaperone proteins that assist in proper folding. Many proteins also undergo post-translational modifications, such as glycosylation or phosphorylation, which further modify their structure and function.
Conclusion: The Polymer Power of Amino Acids
In summary, protein polymers are built from a fundamental set of 20 amino acid monomers. The sequence of these monomers, the primary structure, dictates the higher-order structures (secondary, tertiary, and quaternary) that determine the protein's unique three-dimensional shape and, ultimately, its biological function. The incredible diversity of proteins arises from the vast combinatorial possibilities inherent in arranging 20 amino acids into linear sequences, and their subsequent folding patterns. Understanding the link between amino acid monomers and protein polymers is essential for comprehending the complexity and wonder of biological systems. Further research into protein folding, structure, and function continues to reveal new insights into the workings of life itself.
Latest Posts
Latest Posts
-
When Positioning Your Vehicle In Multilane City Traffic
Apr 11, 2025
-
Gizmos Student Exploration Coral Reefs 1 Abiotic Factors
Apr 11, 2025
-
The Adult Hip Bone Consists Of Regions
Apr 11, 2025
-
The Three Attributes Of Npv Are That It
Apr 11, 2025
-
Which Trait Of Mycenaean Architecture Is Represented In This Image
Apr 11, 2025
Related Post
Thank you for visiting our website which covers about Protein Polymers Are Made Up Of Blank Monomers . We hope the information provided has been useful to you. Feel free to contact us if you have any questions or need further assistance. See you next time and don't miss to bookmark.