The Electrical Properties Of Cells Are The Result Of
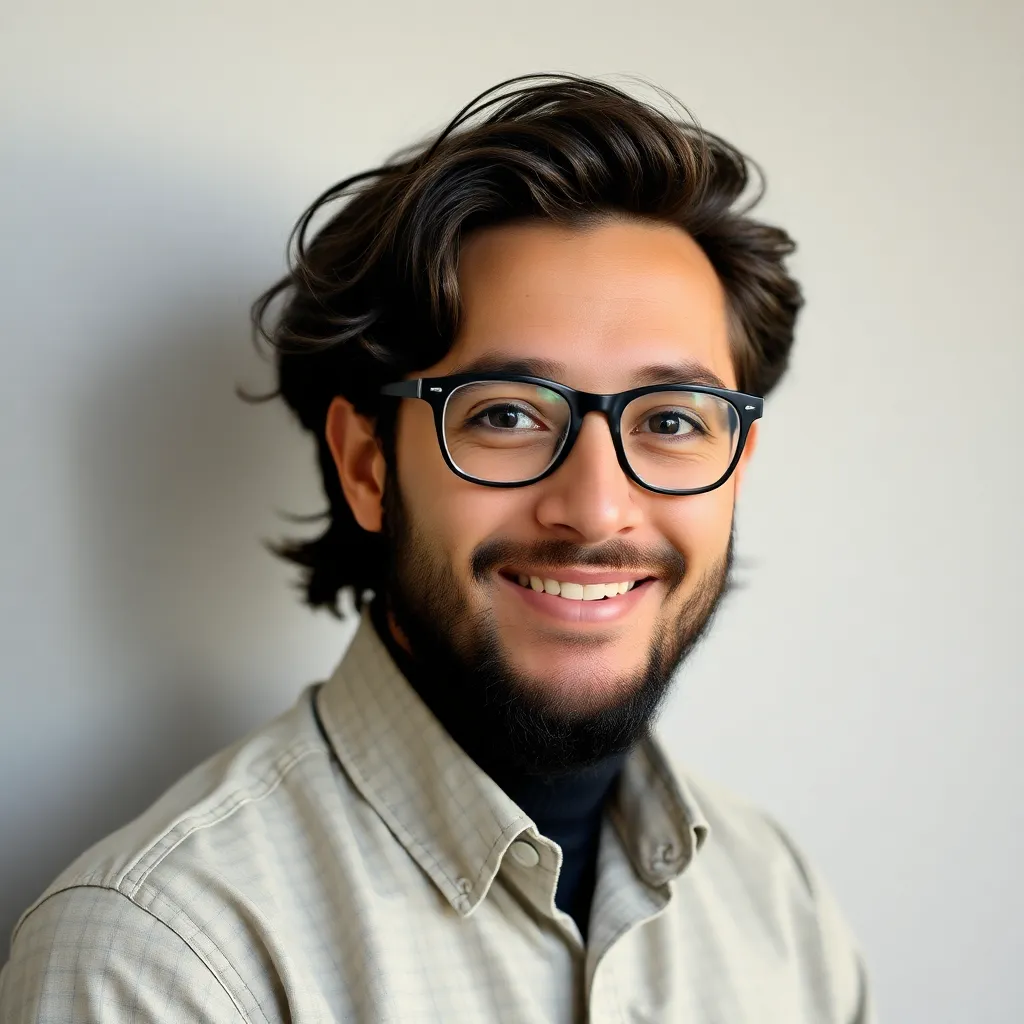
Breaking News Today
May 11, 2025 · 7 min read
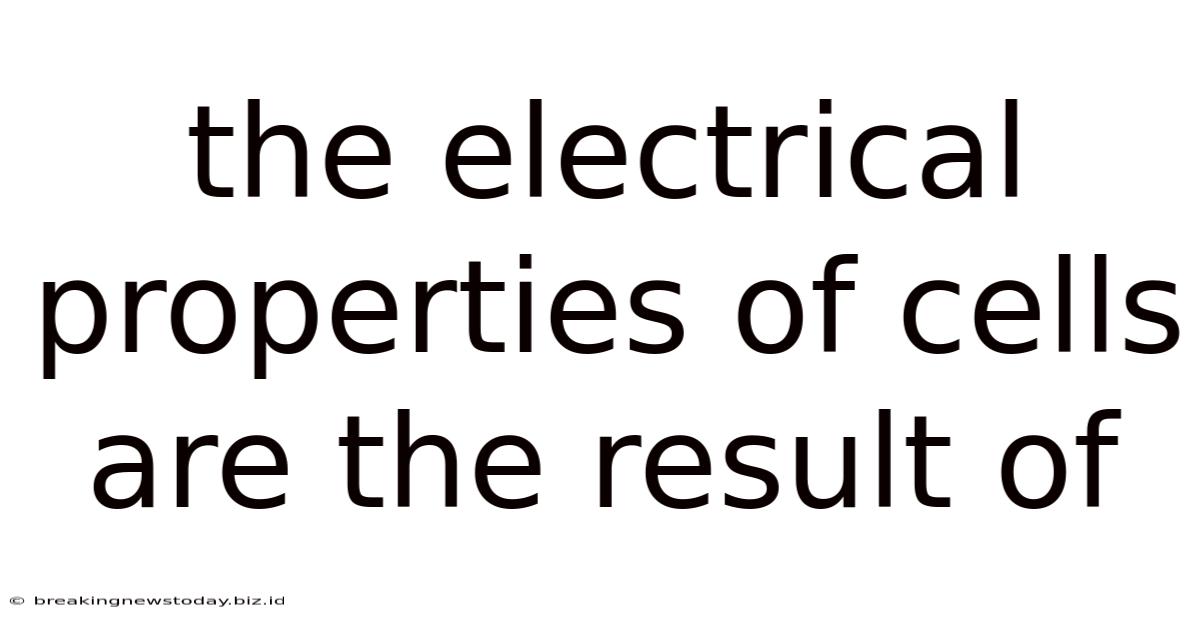
Table of Contents
The Electrical Properties of Cells: A Deep Dive into Ion Channels, Membranes, and Potential
The electrical properties of cells are fundamental to their function, enabling processes as diverse as nerve impulse transmission, muscle contraction, and hormone secretion. These properties aren't magical; they arise from a precisely orchestrated interplay of several key cellular components and mechanisms. This article will delve into the intricacies of cellular electrophysiology, exploring the key players and their roles in establishing and maintaining the electrical potential across cell membranes.
The Cell Membrane: The Foundation of Cellular Electricity
At the heart of cellular electrical activity lies the cell membrane, a selectively permeable lipid bilayer. This structure isn't simply a barrier; it's a dynamic gatekeeper, controlling the flow of ions and molecules into and out of the cell. This control is crucial because it dictates the electrochemical gradient, the difference in both charge and concentration of ions across the membrane. This gradient is the driving force behind many cellular processes.
Lipid Bilayer: A Hydrophobic Heart
The lipid bilayer itself is largely impermeable to charged ions. The hydrophobic tails of phospholipids face inward, forming a barrier that prevents the passage of hydrophilic substances, including most ions. This hydrophobic core is critical in maintaining the separation of charges necessary for electrical potential.
Membrane Proteins: The Gatekeepers
The selectivity of the cell membrane is not solely due to the lipid bilayer; it heavily relies on membrane proteins. These proteins act as channels, carriers, or pumps, facilitating the transport of specific ions across the membrane. Three primary types of membrane proteins contribute significantly to cellular electrical properties:
-
Ion Channels: These integral membrane proteins form pores that allow specific ions (Na+, K+, Ca2+, Cl-) to passively diffuse across the membrane down their electrochemical gradient. They are selective, meaning each channel is typically specific to one or a few types of ions. The opening and closing of ion channels are highly regulated, often responding to changes in voltage (voltage-gated channels), ligand binding (ligand-gated channels), or mechanical stimuli (mechanically-gated channels).
-
Ion Carriers/Transporters: Unlike channels that form continuous pores, carriers bind ions and undergo conformational changes to transport them across the membrane. This process can be passive (facilitated diffusion) or active (requiring energy), often involving co-transport of multiple ions.
-
Ion Pumps: These proteins actively transport ions against their electrochemical gradient, requiring energy in the form of ATP. The most crucial ion pump is the sodium-potassium pump (Na+/K+ ATPase), which maintains the resting membrane potential by pumping three Na+ ions out of the cell and two K+ ions into the cell for every ATP molecule hydrolyzed. This constant pumping creates a significant imbalance in ion concentrations across the membrane, crucial for establishing the resting membrane potential.
The Resting Membrane Potential: The Cell's Baseline Voltage
The resting membrane potential (RMP) is the voltage difference across the cell membrane when the cell is at rest, i.e., not actively generating an electrical signal. This potential is typically negative, ranging from -40 mV to -90 mV, depending on the cell type. This negativity is primarily due to the higher permeability of the membrane to potassium ions (K+) compared to sodium ions (Na+) at rest.
Potassium's Crucial Role
The RMP is largely determined by the equilibrium potential of potassium (EK). At rest, potassium leak channels are open, allowing potassium ions to passively diffuse down their concentration gradient (high inside, low outside). This outward movement of positive charge leaves the inside of the cell relatively negative compared to the outside. The Nernst equation can be used to calculate the equilibrium potential for any given ion, considering its concentration gradient.
Sodium's Contribution & The Pump's Importance
While potassium is the major contributor to the RMP, sodium ions also play a role. Although the membrane is less permeable to sodium at rest, the small inward sodium leak counteracts the outward potassium movement to some extent. The Na+/K+ ATPase pump continuously removes sodium ions and brings potassium ions in, actively reinforcing the ion concentration gradients and maintaining the negative RMP. Without the pump, the gradients would eventually dissipate, and the RMP would disappear.
Action Potentials: Rapid Changes in Membrane Potential
Action potentials are rapid, transient changes in membrane potential that are the basis of electrical signaling in excitable cells like neurons and muscle cells. These potentials are all-or-nothing events, meaning they either occur fully or not at all.
Depolarization: The Rising Phase
An action potential begins with depolarization, a rapid increase in membrane potential from negative to positive. This is triggered when a stimulus causes a sufficient influx of positive ions, typically sodium ions, into the cell. Voltage-gated sodium channels open in response to depolarization, leading to a massive influx of sodium ions and a rapid reversal of the membrane potential.
Repolarization: Returning to Rest
Following depolarization, repolarization occurs. Voltage-gated sodium channels inactivate, and voltage-gated potassium channels open. The outward flow of potassium ions restores the negative membrane potential, returning the cell to its resting state.
Hyperpolarization: A Brief Overshoot
Often, a brief period of hyperpolarization follows repolarization, where the membrane potential becomes more negative than the resting potential. This is due to the continued outward potassium current after the potassium channels close slowly. The Na+/K+ ATPase pump then restores the original ion concentrations and the RMP.
Refractory Periods: Limiting Firing Rate
The refractory period is a period following an action potential during which the cell is less excitable or completely unexcitable. This period is crucial in preventing the generation of continuous action potentials and ensuring the unidirectional propagation of signals.
Propagation of Action Potentials: Spreading the Signal
Action potentials don't remain localized; they propagate along the membrane of excitable cells, transmitting signals over long distances. This propagation relies on the local depolarization of adjacent membrane regions, triggering the opening of voltage-gated sodium channels and the generation of new action potentials.
Myelination: Speeding Up Conduction
In many neurons, the axon is covered in a myelin sheath, a fatty insulating layer produced by glial cells (oligodendrocytes in the CNS and Schwann cells in the PNS). Myelin dramatically increases the speed of action potential propagation by limiting ion flow to the Nodes of Ranvier, the gaps between myelin segments. This "saltatory conduction" allows for rapid transmission of signals over long distances.
Cellular Electrical Properties in Different Cell Types
The specific electrical properties of cells vary significantly depending on their function and type. For example:
-
Neurons: Exhibit high levels of excitability, generating and propagating action potentials rapidly to transmit information throughout the nervous system.
-
Muscle cells: Generate action potentials to initiate muscle contraction. The properties of muscle cell action potentials differ slightly from those of neurons, reflecting their specific contractile function.
-
Cardiac muscle cells: Have unique electrical properties that allow for coordinated heart contractions. Cardiac cells exhibit spontaneous depolarization, initiating rhythmic action potentials that drive the heartbeat.
-
Endocrine cells: Use changes in membrane potential to regulate hormone secretion. Depolarization can trigger the opening of voltage-gated calcium channels, leading to calcium influx and hormone release.
Clinical Significance: Disruptions in Cellular Electricity
Disruptions in the normal electrical properties of cells can have significant clinical consequences. For example:
-
Cardiac arrhythmias: Abnormal heart rhythms are often caused by disturbances in the electrical activity of cardiac muscle cells.
-
Epilepsy: Seizures are caused by abnormal electrical activity in the brain.
-
Muscle disorders: Many muscle diseases involve defects in the electrical properties of muscle cells, leading to weakness or paralysis.
-
Neurological diseases: A wide range of neurological disorders, such as multiple sclerosis, are associated with disruptions in nerve impulse transmission.
Conclusion: A Complex Symphony of Ions and Membranes
The electrical properties of cells are a complex yet elegantly orchestrated phenomenon. The precise interplay of ion channels, pumps, transporters, and the cell membrane itself creates the electrical gradients that are fundamental to life. Understanding these properties is critical not only for advancing our basic biological knowledge but also for developing new therapies for a wide range of diseases. Further research into the intricate mechanisms of cellular electrophysiology continues to reveal new insights into these fundamental processes and their roles in health and disease.
Latest Posts
Related Post
Thank you for visiting our website which covers about The Electrical Properties Of Cells Are The Result Of . We hope the information provided has been useful to you. Feel free to contact us if you have any questions or need further assistance. See you next time and don't miss to bookmark.