The Excitable Cells Of The Nervous System Are Called
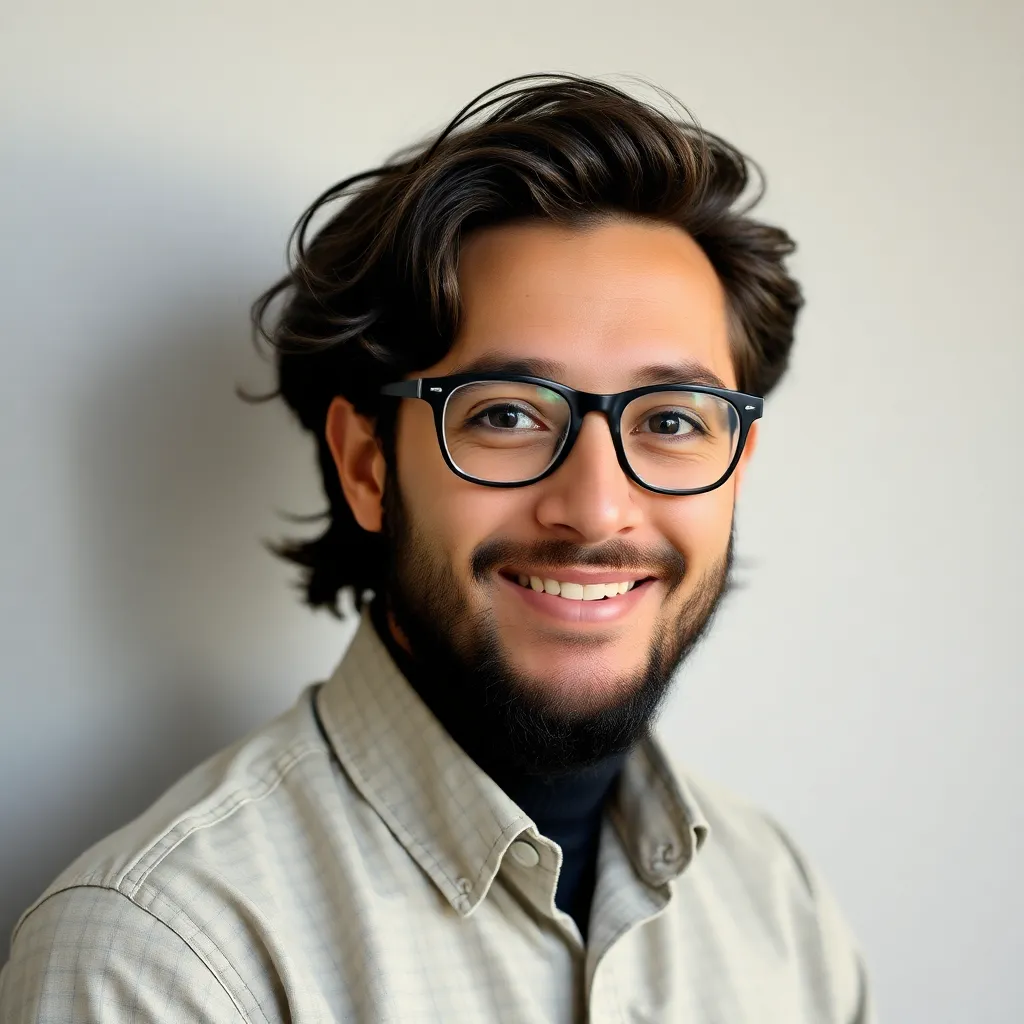
Breaking News Today
Apr 10, 2025 · 8 min read

Table of Contents
The Excitable Cells of the Nervous System are Called Neurons: A Deep Dive into Their Structure, Function, and Significance
The nervous system, a marvel of biological engineering, orchestrates the intricate symphony of our thoughts, actions, and sensations. At the heart of this complex network lie excitable cells, primarily neurons, responsible for receiving, processing, and transmitting information throughout the body. Understanding the structure, function, and significance of these remarkable cells is crucial to comprehending the very essence of what makes us sentient beings.
What are Neurons?
Neurons, also known as nerve cells, are the fundamental units of the nervous system. Their primary function is to transmit information via electrochemical signals. Unlike other cells in the body, neurons possess unique structural and functional properties that enable them to perform this specialized role. This ability to transmit information rapidly and efficiently is what makes them "excitable." Their excitability stems from the ability of their membranes to rapidly change their electrical potential, a process crucial for signal transmission.
The Structure of a Neuron
A typical neuron comprises three main components:
-
Soma (Cell Body): The soma contains the neuron's nucleus and other organelles essential for cellular metabolism and maintenance. It acts as the neuron's metabolic center, synthesizing proteins and maintaining the overall health of the cell.
-
Dendrites: These are branched extensions projecting from the soma. Dendrites act as the primary receptors of neuronal signals. They receive signals from other neurons via specialized junctions called synapses. The extensive branching of dendrites significantly increases the surface area available for receiving signals, allowing a single neuron to integrate input from numerous other neurons. The more dendrites a neuron has, generally the more complex its processing capabilities.
-
Axon: A single, long projection extending from the soma, the axon is responsible for transmitting signals away from the cell body. The axon's length can vary dramatically, ranging from a few micrometers to over a meter in some cases (e.g., those connecting the spinal cord to the toes). The axon is often covered by a myelin sheath, a fatty insulating layer that dramatically speeds up signal transmission. The gaps in the myelin sheath are known as Nodes of Ranvier. At the end of the axon, the signal is transmitted to other neurons or effector cells (e.g., muscle cells) through axon terminals.
Types of Neurons
Neurons exhibit remarkable diversity in terms of their size, shape, and function. This diversity reflects the intricate and specialized roles they play within the nervous system. Some key classifications include:
-
Sensory Neurons (Afferent Neurons): These neurons transmit sensory information from the peripheral nervous system to the central nervous system (brain and spinal cord). They detect stimuli such as touch, temperature, pain, and light, converting them into electrical signals that are relayed to the CNS for processing.
-
Motor Neurons (Efferent Neurons): Motor neurons transmit signals from the CNS to effector organs, such as muscles and glands. These signals trigger muscle contractions or glandular secretions, resulting in movement or physiological changes.
-
Interneurons: These neurons reside entirely within the CNS, acting as intermediaries between sensory and motor neurons. They play a critical role in processing information and integrating signals from multiple sources. The vast majority of neurons in the brain and spinal cord are interneurons. Their complex networks are responsible for the higher-order cognitive functions that define human consciousness.
The Action Potential: The Basis of Neuronal Communication
The key to neuronal function lies in the action potential, a rapid, transient change in the electrical potential across the neuron's membrane. This electrochemical signal forms the basis of neuronal communication, allowing information to be transmitted over long distances.
Stages of the Action Potential
The action potential follows a precise sequence of events:
-
Resting Potential: In its resting state, the neuron's membrane maintains a negative electrical potential (around -70 mV). This is due to an uneven distribution of ions (primarily sodium (Na+) and potassium (K+)) across the membrane, maintained by ion pumps and channels.
-
Depolarization: When a neuron receives sufficient excitatory input, the membrane potential becomes less negative. If the potential reaches a threshold level, voltage-gated sodium channels open, causing a rapid influx of sodium ions into the cell. This leads to a dramatic and rapid depolarization, reversing the membrane potential to a positive value.
-
Repolarization: Following depolarization, voltage-gated potassium channels open, allowing potassium ions to flow out of the cell. This outflow of positive charge restores the negative membrane potential.
-
Hyperpolarization: The outflow of potassium ions often leads to a temporary hyperpolarization, where the membrane potential becomes more negative than the resting potential. This refractory period prevents the immediate firing of another action potential, ensuring the unidirectional propagation of the signal.
-
Return to Resting Potential: Ion pumps gradually restore the resting ion concentrations, returning the membrane potential to its baseline level.
Myelin Sheath and Saltatory Conduction
The myelin sheath, a fatty insulating layer surrounding many axons, significantly increases the speed of action potential propagation. Instead of propagating continuously along the axon, the action potential "jumps" between the Nodes of Ranvier in a process called saltatory conduction. This saltatory conduction is dramatically faster than continuous conduction, allowing for rapid transmission of information throughout the nervous system.
Synaptic Transmission: Communicating Between Neurons
The communication between neurons occurs at specialized junctions called synapses. These synapses are typically formed between the axon terminal of one neuron (the presynaptic neuron) and the dendrite or soma of another neuron (the postsynaptic neuron).
The Process of Synaptic Transmission
-
Neurotransmitter Release: When an action potential reaches the axon terminal, it triggers the release of neurotransmitters, chemical messengers stored in vesicles within the presynaptic neuron.
-
Neurotransmitter Binding: The released neurotransmitters diffuse across the synaptic cleft (the gap between the pre- and postsynaptic neurons) and bind to specific receptors on the postsynaptic membrane.
-
Postsynaptic Potential: The binding of neurotransmitters to their receptors can either depolarize (excitatory postsynaptic potential, EPSP) or hyperpolarize (inhibitory postsynaptic potential, IPSP) the postsynaptic neuron. EPSPs increase the likelihood of the postsynaptic neuron firing an action potential, while IPSPs decrease this likelihood.
-
Signal Integration: A single postsynaptic neuron may receive input from many presynaptic neurons. The neuron integrates these excitatory and inhibitory inputs, determining whether or not it will fire an action potential. This integration process is crucial for complex information processing within the nervous system.
-
Neurotransmitter Removal: After binding to their receptors, neurotransmitters are removed from the synaptic cleft through various mechanisms, including reuptake by the presynaptic neuron, enzymatic degradation, or diffusion. This removal is essential for terminating the signal and preventing continuous stimulation of the postsynaptic neuron.
The Significance of Neurons: Orchestrating Bodily Functions
Neurons are not merely the building blocks of the nervous system; they are the orchestrators of a vast array of bodily functions, from simple reflexes to complex cognitive processes.
Sensory Perception
Sensory neurons are responsible for detecting and transmitting information about our environment, allowing us to experience the world through our senses. These neurons convert physical stimuli (light, sound, pressure, temperature, chemicals) into electrical signals that are relayed to the brain for processing.
Motor Control
Motor neurons control our movements by transmitting signals from the brain and spinal cord to our muscles. Precise coordination of motor neuron activity is essential for everything from walking and talking to fine motor skills such as writing and playing musical instruments.
Cognitive Functions
The complex networks of interneurons within the brain are responsible for higher-order cognitive functions, including thought, memory, language, and emotion. These functions are not localized to specific regions of the brain but rather emerge from the intricate interactions of vast neuronal networks.
Diseases and Disorders Affecting Neurons
A wide range of diseases and disorders can affect the function of neurons, leading to significant neurological impairments. Some key examples include:
-
Alzheimer's Disease: A progressive neurodegenerative disorder characterized by the loss of neurons and the formation of amyloid plaques and neurofibrillary tangles in the brain.
-
Parkinson's Disease: A neurodegenerative disorder affecting motor control, caused by the degeneration of dopamine-producing neurons in the substantia nigra.
-
Multiple Sclerosis (MS): An autoimmune disease affecting the myelin sheath, leading to disruptions in neuronal signaling and causing a variety of neurological symptoms.
-
Stroke: A sudden interruption of blood supply to part of the brain, leading to neuronal death and potential neurological deficits.
Conclusion
The excitable cells of the nervous system, primarily neurons, are the fundamental units of communication within the body. Their unique structure and function enable them to receive, process, and transmit information with remarkable speed and precision. Understanding the intricate workings of neurons is essential to comprehending the complexity of the nervous system and the diverse range of functions it orchestrates. Further research into neuronal function continues to unlock the secrets of the brain and pave the way for new treatments and therapies for neurological disorders. The continued exploration of these fascinating cells remains paramount in advancing our understanding of the human experience and the remarkable power of the nervous system.
Latest Posts
Latest Posts
-
Which Gang Activity Leads To Legal Consequences
Apr 18, 2025
-
Compression Injuries To The Abdomen That Occur During A Motor
Apr 18, 2025
-
Which Is Not A Property Of Metals
Apr 18, 2025
-
Which Phrase From Meeting At Night Contains Alliteration
Apr 18, 2025
-
Compared To Fog Nozzle Streams Smooth Bore Nozzle Streams
Apr 18, 2025
Related Post
Thank you for visiting our website which covers about The Excitable Cells Of The Nervous System Are Called . We hope the information provided has been useful to you. Feel free to contact us if you have any questions or need further assistance. See you next time and don't miss to bookmark.