The Rate At Which Work Is Done Is
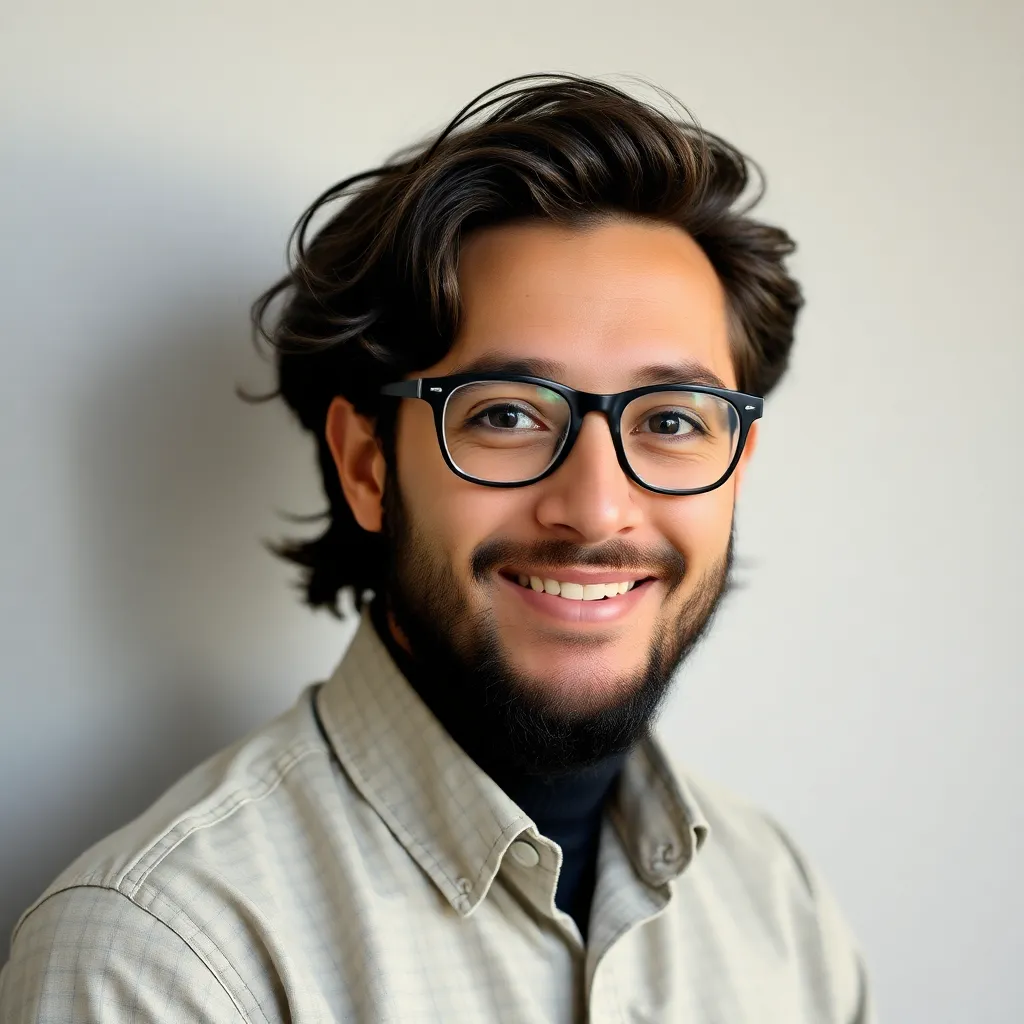
Breaking News Today
Apr 22, 2025 · 6 min read

Table of Contents
The Rate at Which Work is Done: Understanding Power and Efficiency
The rate at which work is done is a fundamental concept in physics and engineering, often expressed as power. Understanding power and its relationship to work and energy is crucial in numerous fields, from designing efficient engines to analyzing human performance. This article delves into the intricacies of power, exploring its definition, various forms, applications, and the factors influencing its calculation. We will also investigate the closely related concept of efficiency, examining how it impacts the rate at which work is accomplished.
Defining Power: The Heart of the Matter
Power, simply put, is the rate at which work is done or energy is transferred. It quantifies how quickly a task is completed or how rapidly energy changes form. Mathematically, power (P) is expressed as:
P = W/t
Where:
- P represents power, typically measured in Watts (W) – one Joule per second (J/s).
- W represents work done, measured in Joules (J).
- t represents the time taken, measured in seconds (s).
This equation tells us that a higher power output means more work is done in less time. A powerful engine, for instance, can complete the same amount of work as a less powerful engine but in a shorter time frame.
Units of Power: A Global Language
While Watts are the most common unit for power, other units are used depending on the context:
- Horsepower (hp): A historical unit still prevalent in automotive and mechanical engineering. 1 hp is approximately equivalent to 746 Watts.
- Kilowatts (kW): A multiple of Watts, frequently used for larger power systems, such as electricity generation and industrial machinery.
- Megawatts (MW): Another multiple of Watts, typically used for extremely large power applications, such as power plants.
Understanding these different units and their conversions is vital for accurate interpretation of power ratings across various fields.
Work and Energy: The Foundation of Power
To fully grasp the concept of power, it's essential to understand the relationship between work and energy. Work, in physics, is done when a force causes an object to move a certain distance in the direction of the force. The formula for work is:
W = Fd cosθ
Where:
- W is work.
- F is the force applied.
- d is the distance moved.
- θ is the angle between the force and the direction of motion.
Energy, on the other hand, is the capacity to do work. Various forms of energy exist, including kinetic energy (energy of motion), potential energy (stored energy), thermal energy (heat), and chemical energy. The principle of conservation of energy states that energy cannot be created or destroyed, only transformed from one form to another.
The connection between work and energy is that doing work involves the transfer or transformation of energy. When work is done on an object, its energy changes. For example, lifting a weight (work) increases its potential energy.
Types and Applications of Power
Power calculations and their applications span a vast range of scenarios. Let's explore some key examples:
Mechanical Power: The Engine of Industry
Mechanical power involves the transfer of energy through mechanical means, such as engines, motors, and machines. Calculating the power of a machine involves measuring the work done and the time taken. Applications range from the power output of a car engine to the power required to lift heavy objects using cranes.
Electrical Power: Powering Our Modern World
Electrical power is the rate at which electrical energy is transferred. It's crucial in our daily lives, powering everything from our homes and offices to industrial facilities. Electrical power is calculated using the formula:
P = IV
Where:
- P is power.
- I is the current (Amperes).
- V is the voltage (Volts).
Understanding electrical power is vital for designing electrical circuits, choosing appropriate appliances, and managing energy consumption.
Hydraulic Power: Harnessing Fluid Pressure
Hydraulic power utilizes the energy of pressurized fluids to perform work. It's widely used in heavy machinery, such as excavators and bulldozers, where immense force is needed. The power of a hydraulic system depends on the pressure and flow rate of the fluid.
Human Power: The Body's Capacity for Work
Even humans generate power, although at a much smaller scale than machines. The power output of a human body depends on factors like muscle strength, endurance, and the type of activity performed. Understanding human power is essential in fields like ergonomics, sports science, and rehabilitation.
Efficiency: Optimizing the Rate of Work
While power measures the rate of work, efficiency measures how effectively this work is accomplished. It's the ratio of useful work output to the total energy input. Mathematically:
Efficiency = (Useful work output / Total energy input) x 100%
A highly efficient system minimizes energy loss during the process, resulting in a higher proportion of input energy being converted into useful work. For example, a highly efficient engine converts a large percentage of the fuel's energy into mechanical power, minimizing waste heat.
Several factors influence efficiency, including:
- Friction: Friction between moving parts reduces efficiency by converting energy into heat.
- Heat loss: Many processes lose energy as heat, especially in engines and power plants.
- Resistance: Electrical resistance in circuits converts electrical energy into heat, lowering efficiency.
- Design flaws: Poor design can lead to energy losses through various mechanisms.
Improving efficiency is crucial for reducing energy consumption, minimizing costs, and reducing environmental impact. Engineers constantly strive to design more efficient systems across all sectors.
Factors Affecting the Rate at Which Work is Done
Numerous factors can influence the rate at which work is done, including:
- Force applied: A larger force generally allows work to be done faster.
- Distance moved: The greater the distance an object is moved, the more time it takes unless the force is increased proportionately.
- Time taken: Reducing the time taken to complete a task increases the power output.
- Type of machine: Different machines have different power capacities and efficiencies.
- Efficiency of the system: Higher efficiency means less energy is wasted, leading to faster work completion.
- External factors: Environmental conditions like temperature or friction can influence power output.
Understanding these factors allows for optimization of processes to maximize the rate at which work is done.
Real-World Examples: Power in Action
Let's consider some real-world examples to illustrate the concept of power:
- A car engine: A powerful car engine can accelerate faster than a less powerful one because it can do more work in less time.
- A crane lifting a weight: A powerful crane can lift heavy objects more quickly than a less powerful one.
- A human runner: A runner's power output determines their speed and endurance.
- A power plant: A large power plant generates a significant amount of power, supplying electricity to a wide area.
- A computer processor: The processing power of a computer determines how quickly it can execute tasks.
These examples showcase the versatility and importance of understanding power in various aspects of life and engineering.
Conclusion: The Significance of Power and Efficiency
The rate at which work is done, as quantified by power, is a fundamental concept with wide-ranging applications. Understanding power and its relationship to work, energy, and efficiency is crucial for designing efficient systems, optimizing processes, and improving performance across diverse fields. From the design of powerful engines to the optimization of human performance, a thorough grasp of power and efficiency is essential for progress and innovation. Continual advancements in technology and engineering strive to enhance both power and efficiency, ultimately shaping a more productive and sustainable future. The quest for higher power output and greater efficiency remains a driving force behind many technological advancements, pushing the boundaries of what's possible and impacting countless aspects of our lives.
Latest Posts
Latest Posts
-
Wrasse Fish And Black Sea Bass Symbiotic Relationship
Apr 22, 2025
-
Which Statement Is True About The Diagram
Apr 22, 2025
-
Data Are Collected On The 35 Students
Apr 22, 2025
-
Who Is The Artist Of The Image Above
Apr 22, 2025
-
Before Releasing Information To The Public Domain
Apr 22, 2025
Related Post
Thank you for visiting our website which covers about The Rate At Which Work Is Done Is . We hope the information provided has been useful to you. Feel free to contact us if you have any questions or need further assistance. See you next time and don't miss to bookmark.