What Can Happen To An Electron When Sunlight Hits It
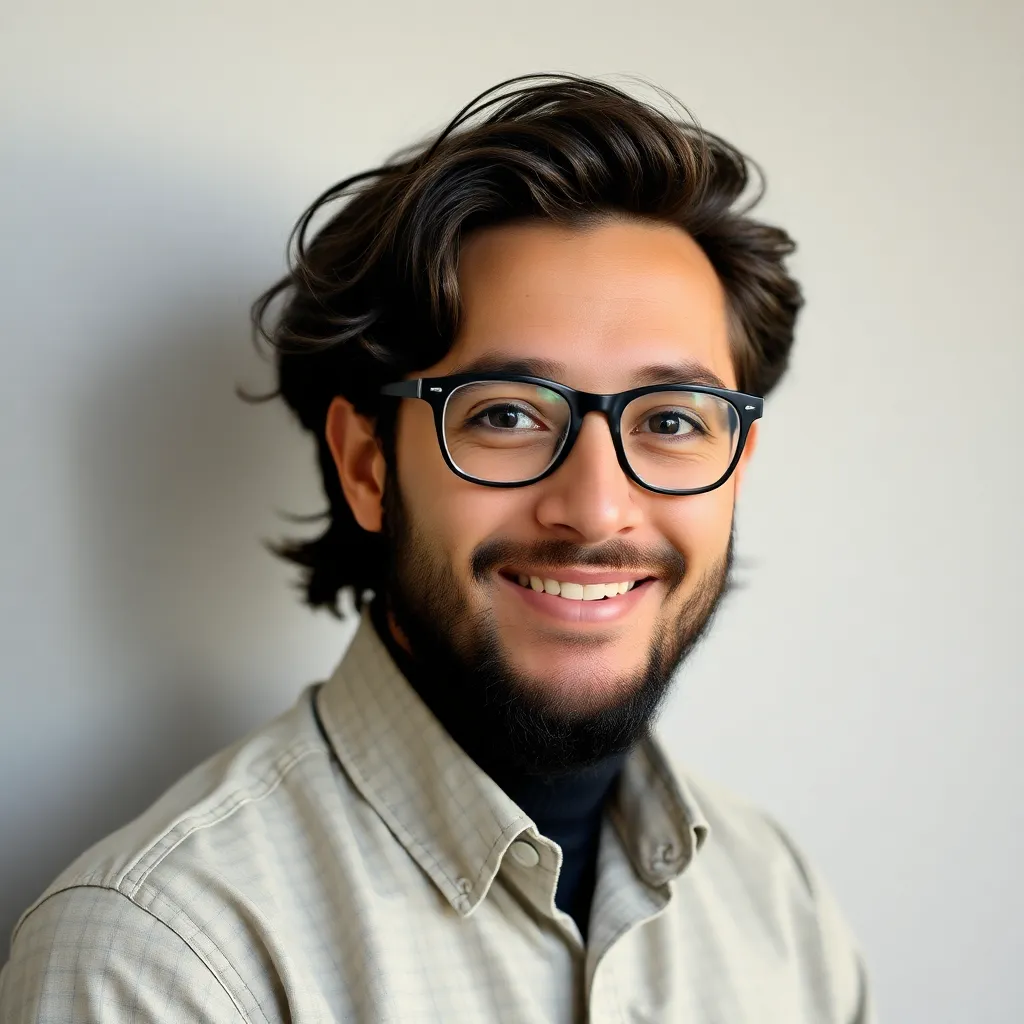
Breaking News Today
May 10, 2025 · 5 min read
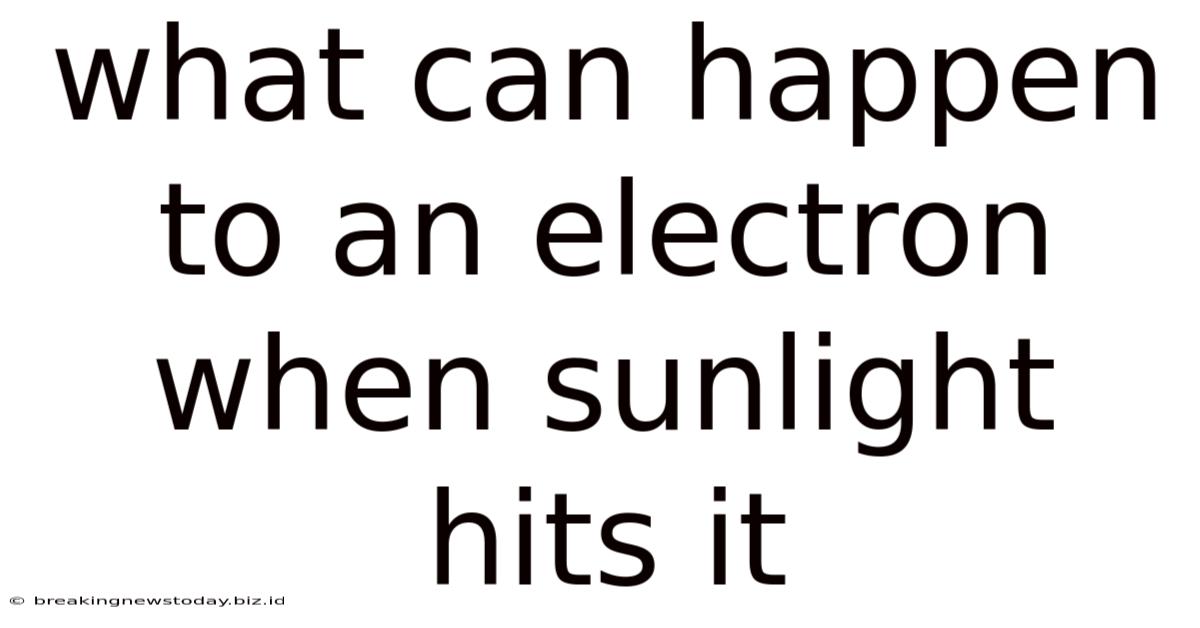
Table of Contents
What Happens When Sunlight Hits an Electron? A Deep Dive into Photoelectric Effects and Beyond
Sunlight, that seemingly benign source of energy, holds a universe of interactions at the atomic level. When sunlight strikes an electron, the consequences depend heavily on the electron's location and the properties of the light itself. This article delves into the fascinating world of light-matter interactions, focusing specifically on what can transpire when a photon of sunlight collides with an electron.
The Photoelectric Effect: A Cornerstone of Quantum Mechanics
One of the most significant consequences of sunlight striking an electron is the photoelectric effect. This phenomenon, pivotal in the development of quantum mechanics, describes the emission of electrons from a material when light shines on it. Crucially, it's not just any light; the light's frequency must be above a certain threshold, known as the work function of the material.
Understanding the Work Function
The work function represents the minimum energy required to liberate an electron from the material's surface. This energy is specific to the material and is related to the strength of the atomic bonds holding the electrons in place. Metals, with their loosely bound valence electrons, typically have lower work functions than non-metals with tightly bound electrons.
The Role of Photons
Sunlight isn't a continuous stream of energy; it's composed of discrete packets of energy called photons. Each photon carries an energy directly proportional to its frequency, described by the equation E = hf, where 'h' is Planck's constant and 'f' is the frequency. Only photons with energy exceeding the material's work function can successfully eject electrons.
The Process of Photoemission
When a photon with sufficient energy strikes an electron, the electron absorbs the photon's energy. If this energy surpasses the work function, the electron gains enough kinetic energy to overcome the attractive forces binding it to the material and escapes. The kinetic energy of the emitted electron is given by:
KE = hf - φ
where φ is the work function. This equation beautifully encapsulates the quantum nature of light and matter interaction.
Beyond the Photoelectric Effect: Other Interactions
While the photoelectric effect is a dominant interaction, it's not the only one. Sunlight contains a broad spectrum of frequencies, and different interactions can occur depending on the photon's energy and the electron's environment.
Absorption and Excitation
Photons with energies below the work function can still interact with electrons. Instead of ejection, these photons can be absorbed by the electrons, causing them to transition to a higher energy level – a process known as excitation. The electron jumps to a higher energy orbital within the atom. This excited state is unstable, and the electron eventually returns to its ground state, releasing the absorbed energy in the form of heat or by emitting a photon of lower energy (fluorescence or phosphorescence).
Compton Scattering: A Change in Momentum
Higher-energy photons in the X-ray and gamma-ray regions of the electromagnetic spectrum can undergo Compton scattering with electrons. In this process, the photon collides with an electron, transferring some of its energy and momentum to the electron. The photon scatters off at a different angle with reduced energy and wavelength, while the electron recoils with increased kinetic energy. This effect demonstrates the particle-like nature of light, as the interaction resembles a collision between two billiard balls.
Pair Production: High-Energy Phenomena
At extremely high energies, such as those found in gamma rays from cosmic sources, a photon can interact with an atomic nucleus, creating an electron-positron pair. This process, known as pair production, requires a photon energy exceeding 1.022 MeV (twice the rest mass energy of an electron). The energy of the photon is converted into the mass of the electron and positron, with any excess energy appearing as kinetic energy of the particles.
The Influence of Material Properties
The response of an electron to sunlight is highly dependent on the material it resides in. Different materials exhibit different electronic structures and optical properties, leading to varied interactions with light.
Conductors vs. Insulators
In conductors, electrons are loosely bound and readily participate in the photoelectric effect or other light-induced processes. In insulators, electrons are tightly bound, requiring significantly higher energy photons for excitation or ejection. This difference explains why metals are often more responsive to light than non-metals.
Semiconductors: The Basis of Modern Electronics
Semiconductors occupy an intermediate position between conductors and insulators. Their electronic properties can be precisely controlled by doping with impurities, making them essential for modern electronics. Sunlight can significantly affect the conductivity of semiconductors, leading to the generation of electron-hole pairs, a process utilized in solar cells to convert sunlight into electricity.
Applications and Technological Implications
The interactions between sunlight and electrons underpin a vast range of technologies crucial to modern life.
Solar Cells: Harnessing the Power of Sunlight
Solar cells, also known as photovoltaic cells, rely on the photoelectric effect to convert sunlight directly into electricity. Sunlight's photons generate electron-hole pairs in a semiconductor material, causing a flow of current. This technology plays an increasingly vital role in clean energy production.
Photodetectors: Sensing Light
Photodetectors utilize the photoelectric effect or other light-matter interactions to detect light. These devices are essential components in various applications, including cameras, optical communication systems, and medical imaging.
Lasers: Precise Light Sources
Lasers operate on the principle of stimulated emission, where excited atoms release photons in a coherent and focused beam. This precise control over light emission has numerous applications in medicine, manufacturing, and telecommunications.
Conclusion: A Microscopic Universe of Interactions
The interaction between sunlight and an electron is far from a simple event. It's a complex phenomenon governed by the principles of quantum mechanics, encompassing the photoelectric effect, absorption and excitation, Compton scattering, and even pair production at high energies. The response of the electron is intricately linked to the properties of the material it's part of and the energy and frequency of the incident photons. Understanding these fundamental interactions is not just a matter of scientific curiosity; it's the foundation for a vast array of technologies shaping our world, from renewable energy to modern electronics and beyond. Further research continues to unveil the subtle nuances of these interactions, promising new discoveries and technological advancements in the future. The ongoing exploration of light-matter interactions remains a frontier of scientific inquiry, with profound implications for technology and our understanding of the universe.
Latest Posts
Latest Posts
-
Latin America Mapping Lab Challenge 4 Answer Key
May 10, 2025
-
Which Of The Following Statements Relating To Supply Is True
May 10, 2025
-
How Many Kilocalories Are Primary Producers For The Ocean Biome
May 10, 2025
-
Translate The Term Rhinitis As Literally As Possible
May 10, 2025
-
If You Are Being Passed You Should
May 10, 2025
Related Post
Thank you for visiting our website which covers about What Can Happen To An Electron When Sunlight Hits It . We hope the information provided has been useful to you. Feel free to contact us if you have any questions or need further assistance. See you next time and don't miss to bookmark.