Which Type Of Tissue Conducts Electrochemical Impulses
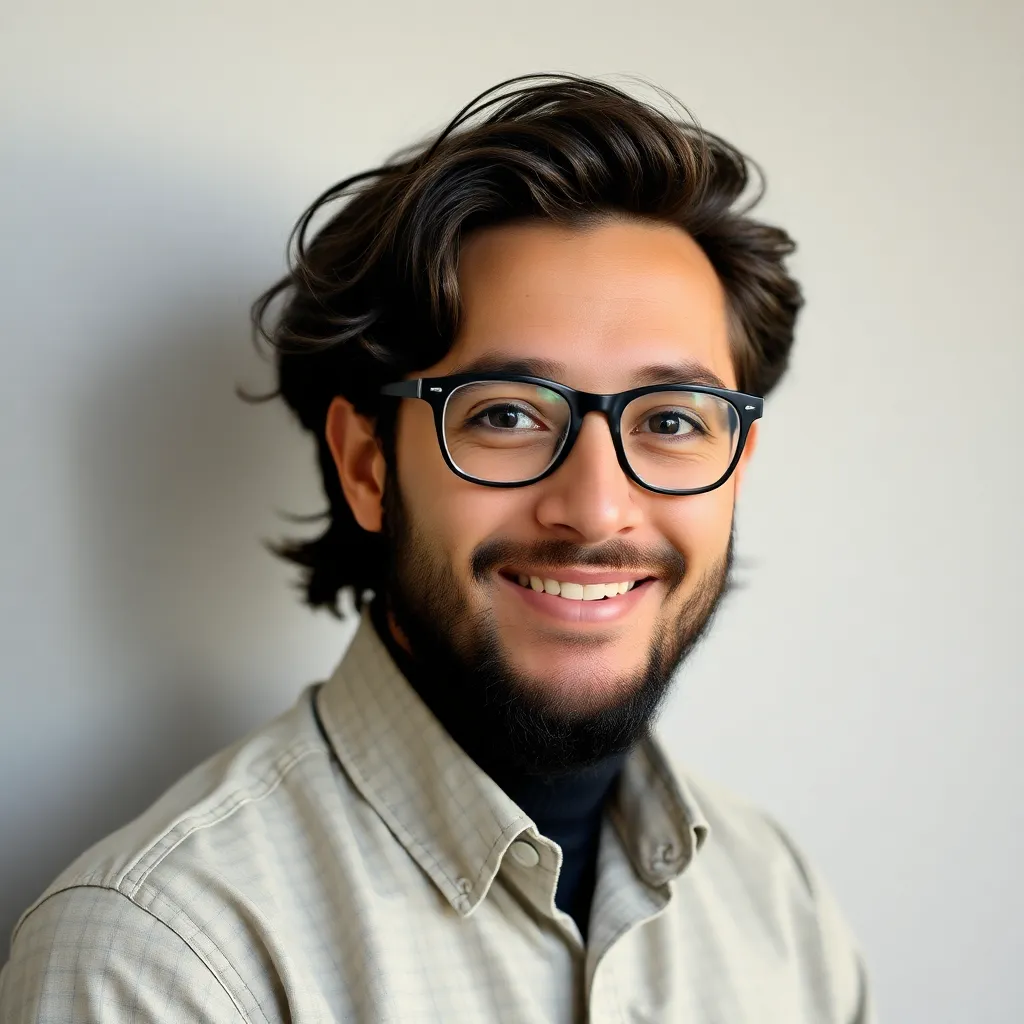
Breaking News Today
May 09, 2025 · 7 min read
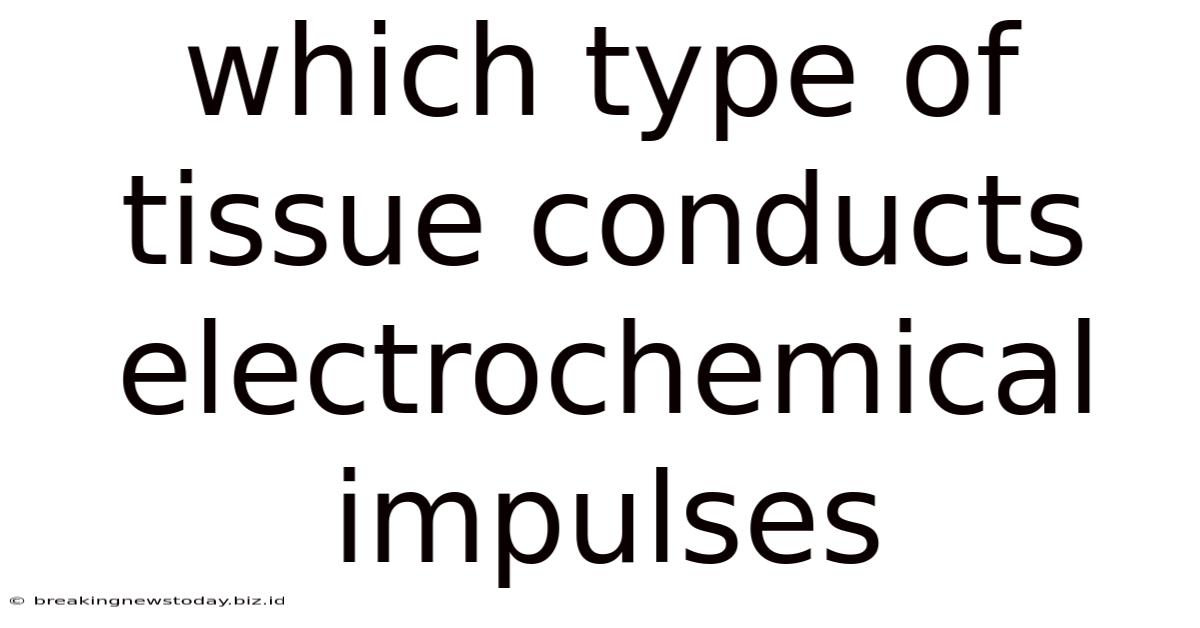
Table of Contents
Which Type of Tissue Conducts Electrochemical Impulses?
Nervous tissue is the only type of tissue in the body that conducts electrochemical impulses. This specialized tissue is responsible for rapid communication throughout the body, allowing for coordinated actions and responses to internal and external stimuli. Understanding the structure and function of nervous tissue, including its unique ability to generate and transmit electrochemical signals, is crucial to grasping the complexities of the human body. This article will delve deep into the intricacies of nervous tissue, exploring its components, mechanisms of electrochemical impulse transmission, and the critical role it plays in maintaining homeostasis and enabling higher-order functions.
The Structure of Nervous Tissue: A Foundation for Electrochemical Signaling
Nervous tissue is primarily composed of two main cell types: neurons and glial cells. These cells work in concert to facilitate the rapid and efficient transmission of electrochemical signals.
Neurons: The Master Communicators
Neurons are the fundamental units of the nervous system, responsible for receiving, processing, and transmitting information. Their unique structure is perfectly adapted for this function. A typical neuron comprises three main parts:
-
Dendrites: These branching extensions receive signals from other neurons or sensory receptors. The large surface area provided by their branching structure maximizes the number of connections a neuron can make. Neurotransmitters, chemical messengers, bind to receptors on the dendrites, initiating the process of electrochemical signaling.
-
Cell Body (Soma): This contains the nucleus and other essential organelles, responsible for maintaining the neuron's metabolism and overall health. The soma integrates incoming signals from the dendrites.
-
Axon: This long, slender projection transmits signals away from the cell body to other neurons, muscles, or glands. The axon is often covered by a myelin sheath, a fatty insulating layer that significantly increases the speed of signal conduction. The myelin sheath is produced by glial cells – oligodendrocytes in the central nervous system and Schwann cells in the peripheral nervous system. The gaps between the myelin sheath segments are called Nodes of Ranvier, which play a crucial role in saltatory conduction (explained later). The axon terminates in axon terminals, also known as synaptic boutons, which release neurotransmitters to communicate with other cells.
Glial Cells: The Unsung Heroes
While neurons are the stars of the show, glial cells, often outnumbering neurons, provide essential support and maintenance for the nervous system. Different types of glial cells perform diverse functions:
-
Astrocytes: These star-shaped cells provide structural support, regulate the extracellular environment, and contribute to the blood-brain barrier. They play a crucial role in maintaining the delicate chemical balance required for proper neuronal function.
-
Oligodendrocytes (CNS) and Schwann Cells (PNS): As mentioned earlier, these cells produce the myelin sheath, crucial for rapid signal transmission.
-
Microglia: These act as the immune cells of the central nervous system, protecting against pathogens and removing cellular debris.
-
Ependymal Cells: These cells line the ventricles of the brain and the central canal of the spinal cord, contributing to the production and circulation of cerebrospinal fluid (CSF).
The Electrochemical Impulse: A Detailed Look
The ability of nervous tissue to conduct electrochemical impulses lies in the unique properties of neuronal membranes. These membranes maintain a difference in electrical potential across them, known as the membrane potential. This potential is primarily established by the unequal distribution of ions, particularly sodium (Na+), potassium (K+), chloride (Cl-), and calcium (Ca2+), across the neuronal membrane.
Resting Membrane Potential: The Silent State
In the resting state, the inside of the neuron is negatively charged relative to the outside. This resting membrane potential, typically around -70 mV, is maintained by several factors:
-
Ion Channels: Specialized protein channels embedded in the neuronal membrane allow specific ions to pass through. These channels are selectively permeable, meaning they only allow certain ions to cross the membrane.
-
Sodium-Potassium Pump: This active transport mechanism actively pumps three sodium ions out of the cell for every two potassium ions pumped in. This creates an unequal distribution of ions, contributing to the negative resting membrane potential.
-
Leak Channels: These channels allow for the passive diffusion of ions across the membrane. Potassium ions leak out of the cell more readily than sodium ions leak in, contributing further to the negative resting membrane potential.
Action Potential: The All-or-None Signal
When a neuron receives a sufficient stimulus, it triggers an action potential, a rapid change in membrane potential that propagates down the axon. This is an all-or-none event; either an action potential occurs, or it doesn't. The strength of the stimulus doesn't affect the amplitude of the action potential, but it can affect the frequency of action potentials.
The action potential involves several distinct phases:
-
Depolarization: A stimulus causes sodium channels to open, allowing sodium ions to rush into the cell. This influx of positive charge rapidly reverses the membrane potential, making the inside of the cell positively charged.
-
Repolarization: Sodium channels close, and potassium channels open, allowing potassium ions to flow out of the cell. This outflow of positive charge restores the negative membrane potential.
-
Hyperpolarization: The outflow of potassium ions can briefly make the membrane potential more negative than the resting potential.
-
Return to Resting Potential: The sodium-potassium pump and leak channels work to restore the resting membrane potential.
Saltatory Conduction: Speeding Up the Signal
In myelinated axons, the action potential doesn't propagate along the entire length of the axon. Instead, it "jumps" from one Node of Ranvier to the next, a process called saltatory conduction. This significantly increases the speed of signal transmission, allowing for rapid communication throughout the nervous system. The myelin sheath acts as an insulator, preventing the leakage of ions and concentrating the depolarization at the Nodes of Ranvier.
The Synapse: Communication Between Neurons
The communication between neurons occurs at specialized junctions called synapses. These junctions typically involve the release of neurotransmitters from the axon terminal of one neuron (the presynaptic neuron) to the dendrites or cell body of another neuron (the postsynaptic neuron).
The process of synaptic transmission involves several steps:
-
Neurotransmitter Release: An action potential reaching the axon terminal triggers the opening of voltage-gated calcium channels, allowing calcium ions to enter the terminal. This influx of calcium triggers the fusion of synaptic vesicles containing neurotransmitters with the presynaptic membrane.
-
Neurotransmitter Diffusion: Neurotransmitters are released into the synaptic cleft, the space between the pre- and postsynaptic neurons.
-
Neurotransmitter Binding: Neurotransmitters diffuse across the synaptic cleft and bind to specific receptors on the postsynaptic membrane. This binding can either excite or inhibit the postsynaptic neuron.
-
Postsynaptic Potential: The binding of neurotransmitters can generate either an excitatory postsynaptic potential (EPSP), causing depolarization and making the postsynaptic neuron more likely to fire an action potential, or an inhibitory postsynaptic potential (IPSP), causing hyperpolarization and making the postsynaptic neuron less likely to fire an action potential.
-
Neurotransmitter Removal: Neurotransmitters are removed from the synaptic cleft through various mechanisms, including reuptake by the presynaptic neuron, enzymatic degradation, or diffusion away from the synapse.
The Importance of Electrochemical Impulses in Bodily Functions
The ability of nervous tissue to conduct electrochemical impulses is fundamental to virtually every aspect of bodily function. Some key examples include:
-
Sensory Perception: Sensory receptors throughout the body detect stimuli (light, sound, touch, temperature, pain) and convert them into electrochemical signals that are transmitted to the brain for processing.
-
Motor Control: The brain sends electrochemical signals to muscles, causing them to contract and generate movement.
-
Autonomic Functions: The autonomic nervous system regulates involuntary functions such as heart rate, breathing, digestion, and blood pressure through electrochemical signals.
-
Cognitive Functions: Complex cognitive processes such as learning, memory, and thought rely on intricate networks of neurons communicating through electrochemical impulses.
-
Hormone Release: The hypothalamus, a part of the brain, controls the release of hormones through electrochemical signals to the pituitary gland.
Conclusion
Nervous tissue, with its unique capacity for electrochemical signaling, stands as a testament to the incredible complexity and sophistication of the human body. The intricate interplay between neurons and glial cells, the precise mechanisms of action potential generation and propagation, and the sophisticated communication at synapses all contribute to the remarkable efficiency and speed of the nervous system. Understanding the properties and functions of nervous tissue provides a foundational understanding of how our bodies function, react to stimuli, and control complex behaviours, highlighting the critical role electrochemical impulses play in maintaining life itself. Further research continues to unravel the intricacies of neuronal communication and its implications for health and disease, paving the way for advancements in neuroscience and medicine.
Latest Posts
Latest Posts
-
The Term That Means Surgical Fixation Of The Spleen Is
May 09, 2025
-
Economists Often Track Employment Trends By Measuring The Proportion
May 09, 2025
-
Why Is Dutch Agriculture Critically Dependent Upon Technology
May 09, 2025
-
The Vertebral Column Encloses And Protects The
May 09, 2025
-
Which Of The Following Is A Polymer
May 09, 2025
Related Post
Thank you for visiting our website which covers about Which Type Of Tissue Conducts Electrochemical Impulses . We hope the information provided has been useful to you. Feel free to contact us if you have any questions or need further assistance. See you next time and don't miss to bookmark.