A Gene Can Best Be Defined As
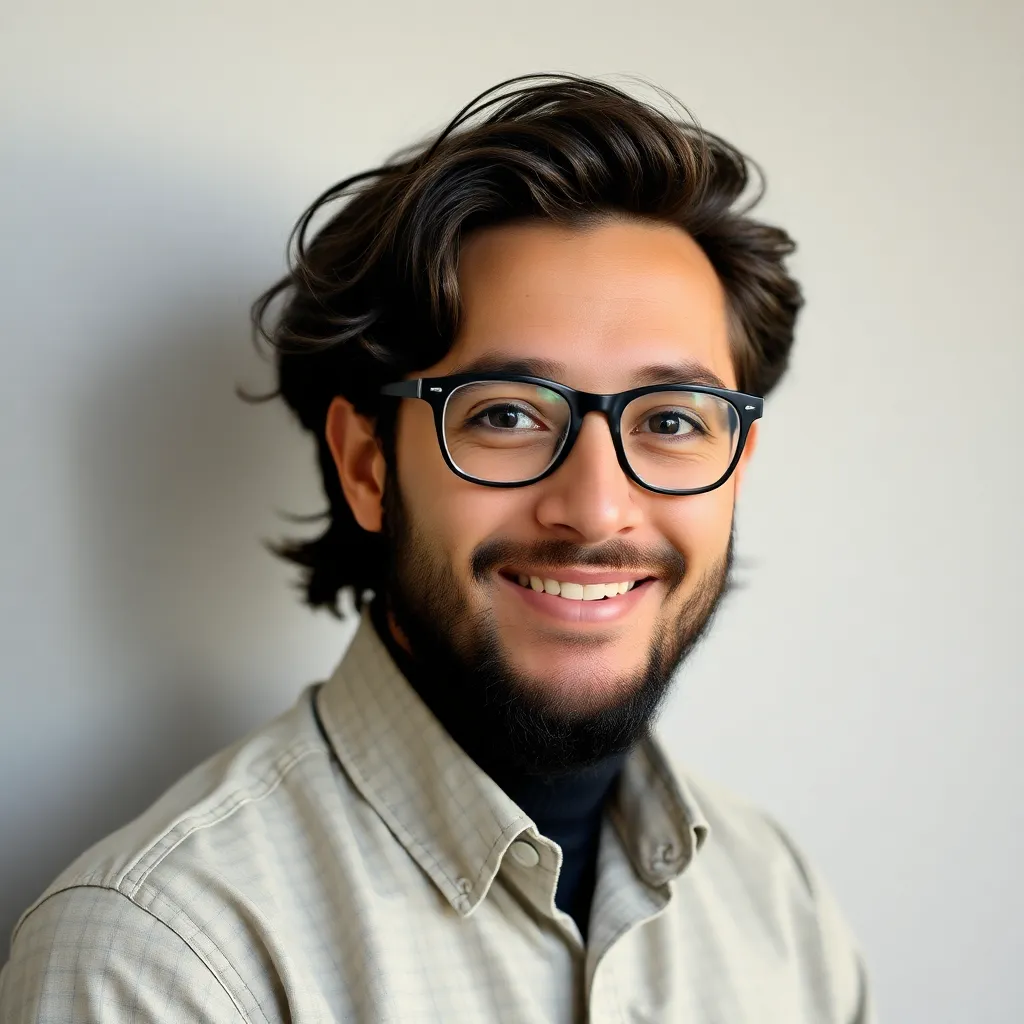
Breaking News Today
Apr 02, 2025 · 7 min read
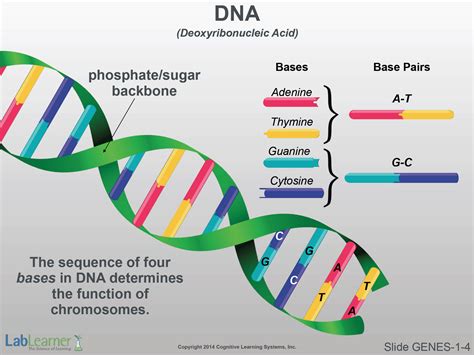
Table of Contents
A Gene Can Best Be Defined As: Exploring the Fundamental Unit of Heredity
A gene, at its most basic level, can be defined as a fundamental unit of heredity. It's a specific sequence of nucleotides within DNA or RNA that is responsible for specifying the synthesis of a single polypeptide chain or functional RNA molecule. However, this seemingly simple definition belies the immense complexity and intricate workings of genes. Understanding what a gene is requires delving into its structure, function, expression, and regulation. This article will explore these aspects in detail, unraveling the intricacies of this fundamental building block of life.
The Structure of a Gene: More Than Just a Sequence
While often depicted as a simple linear sequence, a gene's structure is remarkably nuanced. It's not just a string of DNA or RNA; it's a precisely organized unit with distinct regions crucial for its function. These regions include:
1. Promoters and Enhancers: The On/Off Switches
These regulatory regions don't code for proteins directly but play a vital role in gene expression. Promoters are sequences located upstream of the gene's coding region, serving as binding sites for RNA polymerase, the enzyme responsible for transcription. Enhancers, on the other hand, can be located far upstream or downstream, even on different chromosomes, and enhance the rate of transcription. They act like volume knobs, modulating the level of gene expression. Understanding promoters and enhancers is key to comprehending how genes are turned on or off in different cells and at different times.
2. Exons and Introns: The Coding and Non-coding Regions
Genes contain both coding and non-coding sequences. Exons are the protein-coding segments, transcribed into messenger RNA (mRNA) and eventually translated into proteins. Introns, conversely, are non-coding sequences interspersed within exons. Introns are transcribed into pre-mRNA but are spliced out before translation. This splicing process is a critical step in gene regulation, allowing for alternative splicing, where different combinations of exons are joined together to produce various protein isoforms from a single gene. Alternative splicing vastly expands the proteome, allowing a single gene to code for multiple proteins.
3. 5' and 3' Untranslated Regions (UTRs): Beyond the Coding Sequence
Located at the beginning (5') and end (3') of the mRNA molecule, these untranslated regions are not translated into proteins. However, they play vital roles in mRNA stability, translation efficiency, and localization within the cell. The UTRs are crucial regulatory elements influencing the ultimate amount of functional protein produced from a gene.
4. Polyadenylation Signal: The Termination Signal
This sequence signals the end of transcription and is essential for the addition of a poly(A) tail to the 3' end of the mRNA molecule. The poly(A) tail protects the mRNA from degradation and is crucial for its export from the nucleus and subsequent translation. Without a proper polyadenylation signal, the mRNA would be unstable and unable to produce its corresponding protein.
Gene Function: From DNA to Protein (and Beyond)
The primary function of most genes is to encode proteins. This intricate process involves several key steps:
1. Transcription: DNA to RNA
The first step in gene expression is transcription, where the DNA sequence of a gene is copied into a complementary RNA molecule. RNA polymerase binds to the promoter region and unwinds the DNA double helix. It then synthesizes an RNA molecule using the DNA template strand. The resulting RNA molecule, often mRNA, carries the genetic information encoded in the gene.
2. RNA Processing: Maturation of the mRNA
Before the mRNA can be translated into protein, it undergoes several processing steps. This includes:
- Capping: A modified guanine nucleotide is added to the 5' end of the mRNA, protecting it from degradation and facilitating its binding to ribosomes.
- Splicing: Introns are removed, and exons are joined together to form a mature mRNA molecule.
- Polyadenylation: A poly(A) tail is added to the 3' end of the mRNA, enhancing its stability and translation efficiency.
3. Translation: RNA to Protein
The processed mRNA molecule then travels to the ribosome, the protein synthesis machinery of the cell. Translation is the process of converting the mRNA sequence into a polypeptide chain, using the genetic code. Each three-nucleotide codon in the mRNA specifies a particular amino acid, forming the building blocks of the protein. The polypeptide chain then folds into a specific three-dimensional structure, determining its function.
4. Protein Folding and Modification: Achieving Functionality
Newly synthesized proteins often undergo further modifications, such as glycosylation (addition of sugar molecules) or phosphorylation (addition of phosphate groups), to attain their final functional form. These modifications are crucial for the protein's activity and stability. Incorrect protein folding can lead to various diseases.
Gene Regulation: Controlling Gene Expression
Gene expression is not a constant process; it's tightly regulated to ensure that the right proteins are produced at the right time and in the right amounts. Several mechanisms control gene expression, including:
1. Transcriptional Regulation: Controlling the Initiation of Transcription
This involves the binding of various regulatory proteins to the promoter and enhancer regions, influencing the ability of RNA polymerase to initiate transcription. These proteins can either enhance or repress transcription depending on their function. Transcriptional regulation is a major point of control in gene expression.
2. Post-transcriptional Regulation: Controlling mRNA Processing and Stability
This encompasses regulation at the level of RNA processing, mRNA transport, and mRNA degradation. Alternative splicing, for instance, can produce different protein isoforms from a single gene. mRNA stability is also carefully regulated; some mRNAs are rapidly degraded, while others are relatively stable. Post-transcriptional regulation significantly expands the complexity and versatility of gene expression.
3. Translational Regulation: Controlling Protein Synthesis
Translation can also be controlled, affecting the rate of protein synthesis. Regulatory proteins can bind to the mRNA molecule, influencing its ability to be translated by the ribosome. Translational regulation provides a rapid mechanism to adjust protein levels in response to changing cellular conditions.
4. Post-translational Regulation: Controlling Protein Activity
After protein synthesis, the activity of a protein can be further modulated by various mechanisms, such as phosphorylation, ubiquitination (marking proteins for degradation), and proteolytic cleavage (cutting the protein into smaller fragments). Post-translational regulation is crucial for fine-tuning protein function and ensuring accurate cellular responses.
Beyond Protein Coding Genes: Non-coding RNAs and More
Not all genes encode proteins. A significant portion of the genome is transcribed into non-coding RNAs (ncRNAs), which have diverse functions in gene regulation and other cellular processes. Examples include:
- microRNAs (miRNAs): These small RNAs regulate gene expression by binding to target mRNAs, leading to their degradation or translational repression.
- long non-coding RNAs (lncRNAs): These longer ncRNAs are involved in a wide array of cellular processes, including chromatin remodeling, gene regulation, and X-chromosome inactivation.
- ribosomal RNAs (rRNAs) and transfer RNAs (tRNAs): These are essential components of the protein synthesis machinery.
Gene Mutations and Their Consequences
Changes in the DNA sequence of a gene, known as mutations, can alter its function. These mutations can range from single nucleotide changes (point mutations) to large-scale chromosomal rearrangements. Mutations can have various consequences, depending on their location and nature:
- Silent mutations: These changes don't alter the amino acid sequence of the encoded protein.
- Missense mutations: These changes result in a single amino acid substitution in the protein.
- Nonsense mutations: These changes create a premature stop codon, resulting in a truncated and often non-functional protein.
- Frameshift mutations: These changes alter the reading frame of the gene, leading to a completely different amino acid sequence downstream of the mutation.
Mutations can lead to various diseases, including genetic disorders, cancer, and other complex traits. Understanding the effects of mutations is crucial for developing diagnostic tools and therapeutic strategies.
Gene Therapy and Genetic Engineering: Harnessing the Power of Genes
The understanding of gene structure, function, and regulation has opened doors to groundbreaking technologies like gene therapy and genetic engineering. Gene therapy aims to correct defective genes to treat genetic disorders, while genetic engineering involves modifying genes to enhance the characteristics of organisms. These fields hold immense potential for treating diseases and improving agricultural practices, amongst other things.
Conclusion: The Ever-Evolving Understanding of Genes
The definition of a gene has evolved significantly since the early days of genetics. What began as a simple concept of a unit of heredity has transformed into a multifaceted understanding of intricate regulatory mechanisms, diverse molecular structures, and their impact on human health and disease. Ongoing research continues to uncover new layers of complexity and functionality, solidifying the gene's position as the cornerstone of life itself. From understanding basic inheritance patterns to developing cutting-edge therapeutic strategies, the study of genes remains a pivotal area of scientific inquiry, shaping our understanding of biology and impacting our future.
Latest Posts
Latest Posts
-
The Most Popular Linux Platform For Mobile Phones Is
Apr 03, 2025
-
Mosses Are Located In Which Zone Of Deciduous Forests
Apr 03, 2025
-
How Does Co2 Level Affect Oxygen Production
Apr 03, 2025
-
Ohio Cosmetology State Board Exam Practice Test
Apr 03, 2025
-
Which Type Of Address Is The Ip Address 198 162 12 254 24
Apr 03, 2025
Related Post
Thank you for visiting our website which covers about A Gene Can Best Be Defined As . We hope the information provided has been useful to you. Feel free to contact us if you have any questions or need further assistance. See you next time and don't miss to bookmark.