The Pairing Of Nitrogenous Bases In Dna Is Specific Because
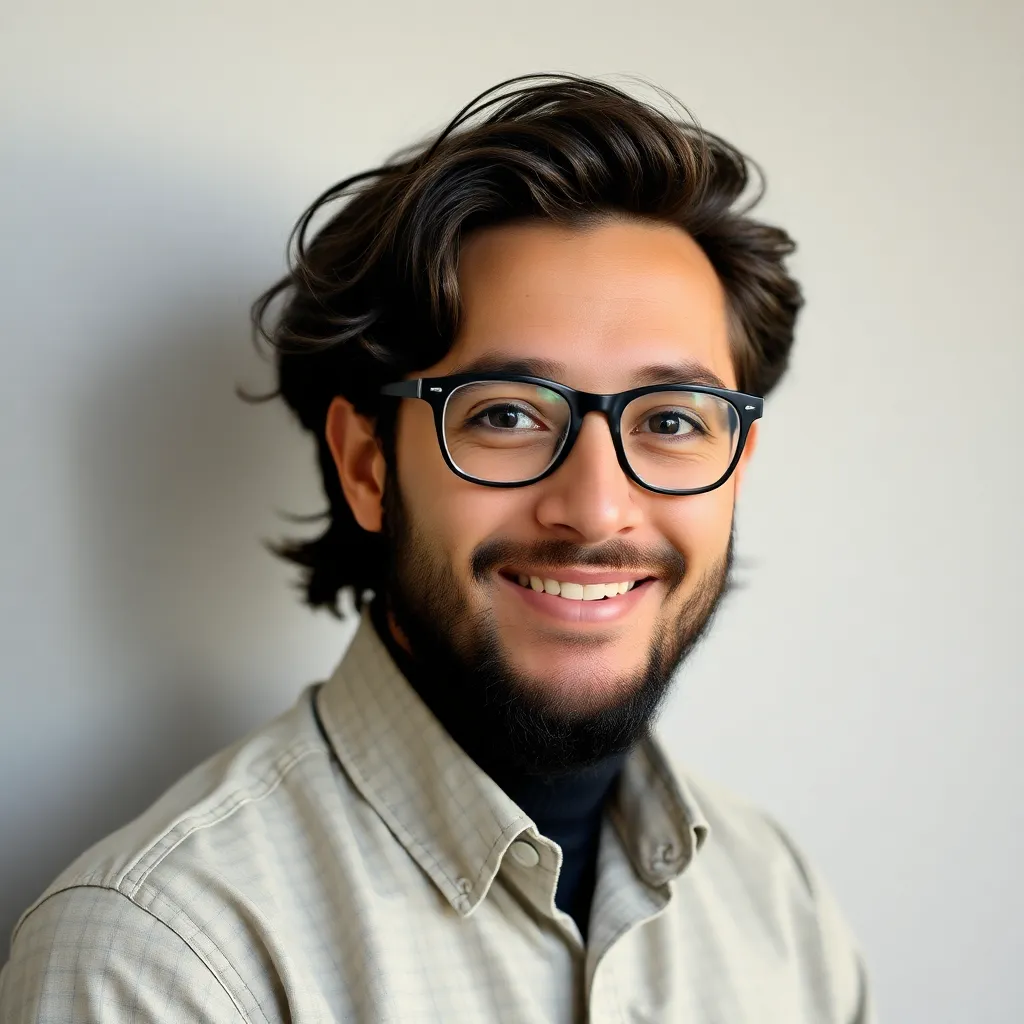
Breaking News Today
Apr 03, 2025 · 6 min read

Table of Contents
The Pairing of Nitrogenous Bases in DNA is Specific Because…
The elegance of life lies, in part, within the seemingly simple yet profoundly intricate structure of DNA. Deoxyribonucleic acid, the blueprint of life, owes its remarkable ability to store and transmit genetic information to the highly specific pairing of its nitrogenous bases. This specificity isn't merely a quirk of nature; it's fundamental to DNA's function, replication, and repair mechanisms. Understanding why this pairing is specific unlocks a deeper appreciation of the molecular machinery driving evolution and heredity.
The Players: Adenine, Guanine, Cytosine, and Thymine
Before delving into the reasons behind base pairing specificity, let's briefly revisit the key players: the four nitrogenous bases – adenine (A), guanine (G), cytosine (C), and thymine (T). These molecules, categorized into purines (A and G, with a double-ring structure) and pyrimidines (C and T, with a single-ring structure), are the fundamental building blocks of DNA's genetic code. Their precise arrangement determines the sequence of genes, dictating the production of proteins and influencing virtually every aspect of an organism's phenotype.
Hydrogen Bonding: The Key to Specificity
The specificity of base pairing arises primarily from hydrogen bonding. Hydrogen bonds are weak electrostatic attractions between a hydrogen atom covalently bonded to a highly electronegative atom (like oxygen or nitrogen) and another electronegative atom. While individually weak, the cumulative effect of multiple hydrogen bonds provides stability to the DNA double helix.
Adenine-Thymine (A-T) Pairing: Two Hydrogen Bonds
Adenine and thymine form a base pair through two hydrogen bonds. Specifically, a hydrogen atom on the amino group of adenine forms a hydrogen bond with an oxygen atom on the carbonyl group of thymine. Another hydrogen bond forms between a hydrogen atom on the nitrogen atom of adenine and a nitrogen atom on thymine. This precise arrangement ensures that only adenine can form a stable pair with thymine, and vice versa. Any other combination would result in an unstable and incorrect pairing, disrupting the DNA's structural integrity.
Guanine-Cytosine (G-C) Pairing: Three Hydrogen Bonds
Guanine and cytosine form a base pair through three hydrogen bonds, making this pairing even stronger than the A-T pairing. A hydrogen bond forms between a nitrogen atom on guanine and an oxygen atom on cytosine. Another bond forms between a nitrogen atom on guanine and a nitrogen atom on cytosine. A third hydrogen bond forms between an oxygen atom on guanine and a hydrogen atom on an amino group of cytosine. The added hydrogen bond further enhances the stability of the G-C base pair. The geometry and arrangement of these hydrogen bond acceptors and donors are crucial for the stability of the base pair. Any deviation would result in steric clashes that destabilize the structure.
Steric Hindrance: Size Matters
Beyond hydrogen bonding, steric hindrance plays a crucial role in the specificity of base pairing. Purines (A and G) are larger molecules than pyrimidines (C and T). The combination of a purine with a pyrimidine is geometrically ideal for maintaining the uniform width of the DNA double helix. Attempting to pair two purines together would result in a bulky, distorted helix, while pairing two pyrimidines would leave a narrow gap, disrupting the helix's overall structure. This structural constraint further reinforces the A-T and G-C pairing preferences.
The Role of Base Stacking
Another significant contributor to DNA's stability is base stacking. The planar aromatic rings of the nitrogenous bases stack upon each other within the DNA double helix, creating hydrophobic interactions (repulsion of water) that contribute significantly to the overall stability of the molecule. This stacking is optimized by the specific purine-pyrimidine pairing, further reducing the likelihood of incorrect pairings.
The Significance of Specificity: Replication and Repair
The precise and specific nature of base pairing is paramount for the faithful replication of DNA. During replication, the DNA double helix unwinds, and each strand serves as a template for the synthesis of a new complementary strand. The specific pairing rules ensure that each new strand is an exact copy of the original strand, minimizing errors and maintaining the integrity of the genetic information. Any deviation from these rules during replication can lead to mutations, potentially with severe consequences for the organism.
Similarly, DNA repair mechanisms rely heavily on base pairing specificity. When DNA damage occurs, such as through exposure to UV radiation or chemical mutagens, repair enzymes utilize the specific base pairing rules to identify and correct errors. They recognize the incorrect base pairings and replace them with the correct ones, preserving the integrity of the genetic code.
Evolutionary Implications
The specificity of base pairing isn't merely a functional necessity; it has played a crucial role in the evolution of life itself. The stability and reliability of DNA replication, ensured by specific base pairing, have allowed for the accurate transmission of genetic information across generations. This fidelity of replication allows for the accumulation of beneficial mutations over time, providing the raw material for natural selection and driving the evolution of diverse life forms. Variations in the DNA sequence, while critical for adaptation, must be tightly regulated to prevent runaway errors that would destabilize the genome.
Beyond the Textbook: Exceptions and Nuances
While A-T and G-C base pairing is the dominant rule, some exceptions and nuances exist. Certain modified bases found in some organisms can form alternative pairings. Also, within specific regions of the DNA, non-canonical base pairing might transiently occur, particularly during DNA replication or repair processes. These exceptions, however, are rare and usually serve specific functions, and do not challenge the fundamental principle of highly specific base pairing that underpins DNA’s structure and function.
Conclusion: A Foundation of Life
The specific pairing of nitrogenous bases in DNA is not just a chemical detail; it's the very foundation of life itself. The precise interactions mediated by hydrogen bonding, steric hindrance, and base stacking ensure the faithful replication and repair of genetic information, allowing for the stability and evolution of life on Earth. Understanding the "why" behind this specificity provides insights into the intricate molecular mechanisms that shape the biological world. The elegance and precision of this molecular system serves as a testament to the power and beauty of natural selection and the evolution of molecular mechanisms. Further research continues to refine our understanding of DNA’s complexities, uncovering subtle variations and new nuances to this fundamental building block of life. The more we learn, the more we appreciate the remarkable precision and elegance of this vital system.
Latest Posts
Latest Posts
-
Factor Analysis Has Been Used To Identify The Most Basic
Apr 04, 2025
-
The Party That Raised The Most Money Overall Is
Apr 04, 2025
-
A Weakness Or Slight Muscular Paralysis Is Known As
Apr 04, 2025
-
Which Is An Example Of A Risky Situation
Apr 04, 2025
-
What Did Extending The Franchise In The United States Mean
Apr 04, 2025
Related Post
Thank you for visiting our website which covers about The Pairing Of Nitrogenous Bases In Dna Is Specific Because . We hope the information provided has been useful to you. Feel free to contact us if you have any questions or need further assistance. See you next time and don't miss to bookmark.