The Sliding Filament Model Of Contraction Involves
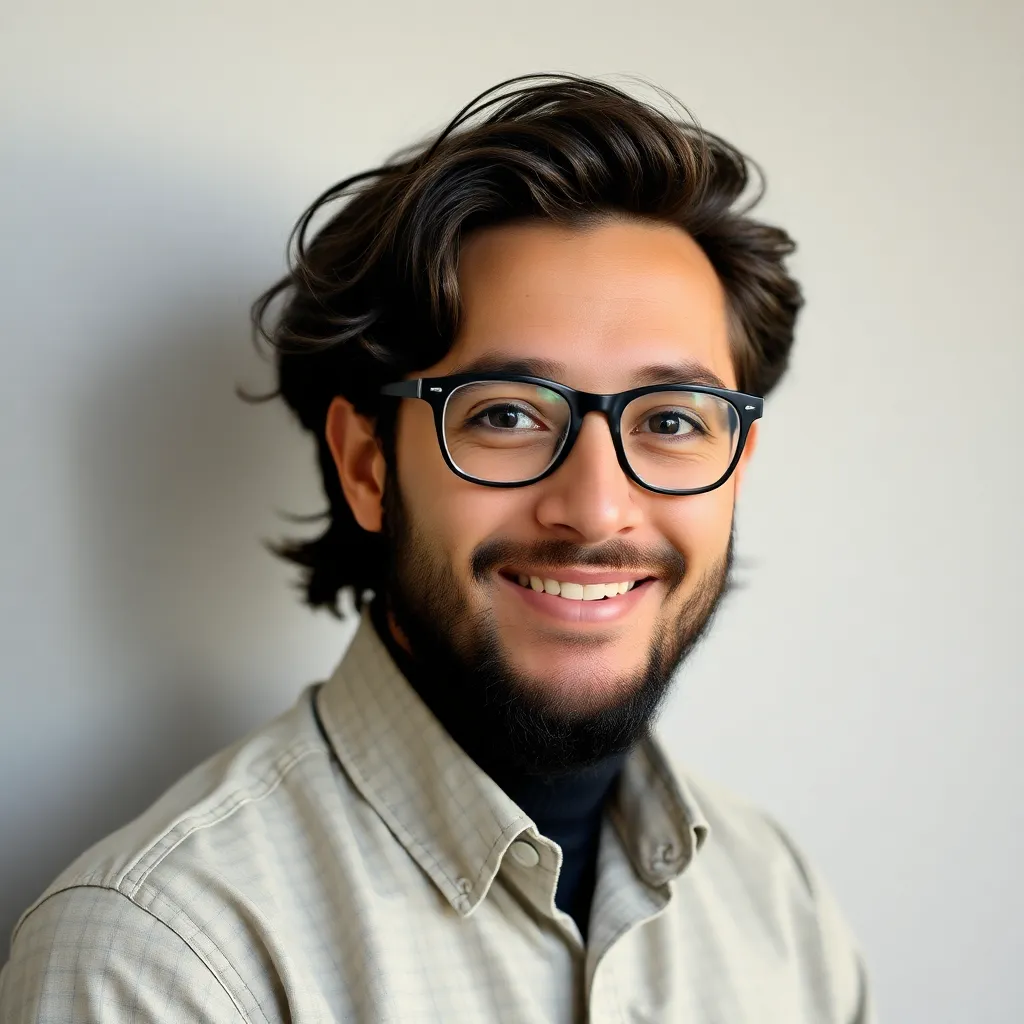
Breaking News Today
May 10, 2025 · 6 min read
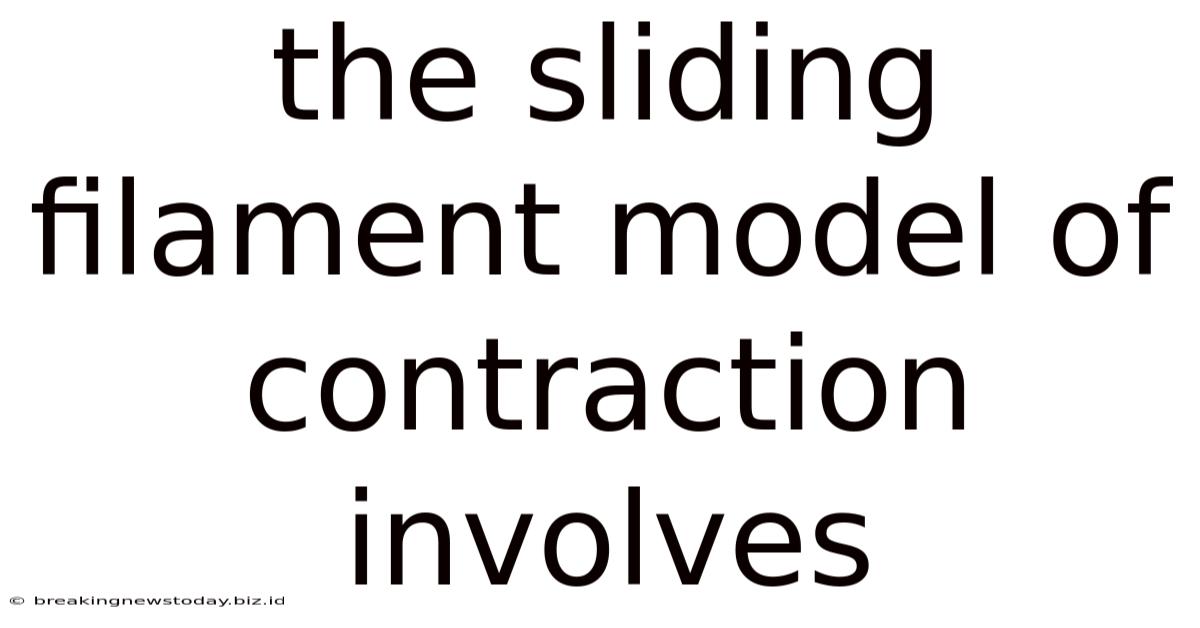
Table of Contents
The Sliding Filament Model of Contraction: A Deep Dive
The sliding filament theory is a cornerstone of our understanding of muscle contraction. It elegantly explains how the seemingly simple act of muscle shortening is achieved through the complex interplay of proteins within muscle fibers. This article will delve deep into the mechanics of this model, exploring the key players, the intricate steps involved, and the regulatory mechanisms that control the process. We'll also touch upon the differences between different muscle types and the implications for various physiological functions.
The Key Players: Actin and Myosin
At the heart of the sliding filament model lie two primary proteins: actin and myosin. These proteins are arranged in a highly organized manner within the sarcomere, the basic contractile unit of muscle.
Actin Filaments: The Thin Filaments
Actin filaments, also known as thin filaments, are composed of two intertwined strands of actin monomers. Associated with these actin strands are two other important proteins:
- Tropomyosin: This long, fibrous protein wraps around the actin filament, covering the myosin-binding sites on actin in a relaxed muscle. This prevents unwanted interaction between actin and myosin.
- Troponin: This protein complex, comprised of three subunits (troponin I, troponin T, and troponin C), sits at intervals along the tropomyosin molecule. Troponin C binds calcium ions, which is crucial for initiating muscle contraction.
Myosin Filaments: The Thick Filaments
Myosin filaments, or thick filaments, are primarily composed of numerous myosin molecules. Each myosin molecule has a head region and a tail region. The myosin heads possess ATPase activity, which means they can hydrolyze ATP (adenosine triphosphate) to release energy. This energy is essential for the power stroke during muscle contraction. The myosin heads are also capable of binding to actin.
The Steps of Muscle Contraction: A Detailed Look
The sliding filament model explains how muscle contraction occurs through a cyclical process involving the interaction of actin and myosin filaments. This process can be broken down into several key steps:
1. Arrival of the Action Potential and Calcium Release:
Muscle contraction is initiated by a nerve impulse, or action potential, arriving at the neuromuscular junction. This triggers the release of acetylcholine, a neurotransmitter, which depolarizes the muscle fiber membrane. This depolarization spreads through the T-tubules, specialized invaginations of the sarcolemma (muscle cell membrane), into the interior of the muscle fiber. This ultimately leads to the release of calcium ions (Ca²⁺) from the sarcoplasmic reticulum (SR), a specialized intracellular calcium store.
2. Calcium Binding to Troponin C:
The released calcium ions bind to troponin C. This binding causes a conformational change in the troponin-tropomyosin complex, which moves tropomyosin away from the myosin-binding sites on the actin filaments. This exposes the binding sites, making them available for interaction with myosin heads.
3. Cross-Bridge Formation:
With the myosin-binding sites now exposed, the myosin heads can bind to actin, forming a cross-bridge. This binding is facilitated by the hydrolysis of ATP, providing the energy needed for the cross-bridge formation.
4. The Power Stroke:
Once the cross-bridge is formed, the myosin head undergoes a conformational change, pivoting and pulling the actin filament towards the center of the sarcomere. This movement is known as the power stroke. ADP and inorganic phosphate (Pi) are released during this step.
5. Cross-Bridge Detachment:
Following the power stroke, a new ATP molecule binds to the myosin head. This binding causes the myosin head to detach from the actin filament.
6. ATP Hydrolysis and Myosin Head Reactivation:
The ATP molecule bound to the myosin head is then hydrolyzed into ADP and Pi. This hydrolysis process resets the myosin head to its high-energy conformation, ready to bind to another actin filament and repeat the cycle.
7. Relaxation:
Muscle relaxation occurs when the nerve impulses cease. This causes the release of calcium from troponin C. Calcium is then actively pumped back into the sarcoplasmic reticulum by calcium ATPase pumps. With calcium removed, troponin-tropomyosin complex returns to its original position, blocking the myosin-binding sites on actin. This prevents further cross-bridge cycling, resulting in muscle relaxation.
Types of Muscle Contractions: Isometric and Isotonic
The sliding filament mechanism underlies two main types of muscle contractions:
-
Isometric contractions: In these contractions, the muscle length remains constant, while the muscle tension increases. A classic example is holding a weight in a fixed position. The muscle fibers are actively generating force, but the opposing force prevents shortening.
-
Isotonic contractions: In these contractions, the muscle tension remains constant, while the muscle length changes. These can be further divided into concentric contractions (muscle shortens) and eccentric contractions (muscle lengthens). Lifting a weight is a concentric isotonic contraction, while slowly lowering the weight is an eccentric isotonic contraction.
Muscle Fiber Types and Their Implications
Muscle fibers can be classified into different types based on their contractile and metabolic properties:
-
Type I (Slow-twitch) fibers: These fibers are slow to contract but resistant to fatigue. They are rich in mitochondria and myoglobin, making them ideal for endurance activities.
-
Type IIa (Fast-twitch oxidative) fibers: These fibers contract faster than Type I fibers and have a moderate resistance to fatigue. They possess a combination of oxidative and glycolytic metabolic capabilities.
-
Type IIx (Fast-twitch glycolytic) fibers: These fibers contract rapidly but fatigue quickly. They rely primarily on glycolysis for energy production.
The proportion of different fiber types varies across individuals and depends on factors such as genetics and training.
The Role of ATP in Muscle Contraction
ATP plays a vital role in all stages of muscle contraction:
-
Cross-bridge formation: ATP hydrolysis provides the energy for the cross-bridge formation between actin and myosin.
-
Power stroke: The energy released during ATP hydrolysis drives the power stroke, pulling the actin filaments towards the center of the sarcomere.
-
Cross-bridge detachment: ATP binding to the myosin head is necessary for cross-bridge detachment.
-
Calcium pump: ATP is also crucial for the active transport of calcium ions back into the sarcoplasmic reticulum, which is essential for muscle relaxation.
Clinical Significance of Understanding the Sliding Filament Model
A comprehensive understanding of the sliding filament model is crucial in various clinical settings:
-
Muscle diseases: Many muscle diseases, such as muscular dystrophy and myasthenia gravis, affect the proteins involved in the sliding filament mechanism. Understanding these disruptions helps in developing effective diagnostic and therapeutic strategies.
-
Rehabilitation and sports medicine: Knowledge of muscle contraction mechanisms is essential in designing effective rehabilitation programs and training regimens for athletes.
-
Pharmacology: Several drugs, such as muscle relaxants and calcium channel blockers, act by influencing the steps involved in the sliding filament process.
-
Understanding aging: The efficiency of the sliding filament mechanism decreases with age, leading to age-related muscle weakness and loss of function. Understanding the mechanisms involved can contribute to strategies aimed at delaying or reversing these age-related changes.
Future Research and Emerging Concepts
While the sliding filament model provides a robust framework for understanding muscle contraction, ongoing research continues to refine and expand our knowledge. Areas of current investigation include:
-
The precise mechanisms involved in regulating calcium release and reuptake.
-
The roles of other proteins and molecules in modulating muscle contraction.
-
The development of novel therapeutic strategies targeting specific aspects of the sliding filament mechanism.
In conclusion, the sliding filament model is a fundamental concept in physiology and a cornerstone of our understanding of how muscles contract. Its intricate mechanisms, the interplay of different proteins, and its clinical implications make it a continuously evolving area of research. Future advancements in this field promise to unlock new avenues for treatment and prevention of muscle-related disorders, as well as enhance our understanding of human movement and athletic performance.
Latest Posts
Latest Posts
-
What Can Happen To An Electron When Sunlight Hits It
May 10, 2025
-
A Difference Between Babur And Akbar Was That
May 10, 2025
-
Summary Of Chapter 1 In Animal Farm
May 10, 2025
-
Implicit Bias Is Characterized By The
May 10, 2025
-
Which Of The Following Is Part Of The Endomembrane System
May 10, 2025
Related Post
Thank you for visiting our website which covers about The Sliding Filament Model Of Contraction Involves . We hope the information provided has been useful to you. Feel free to contact us if you have any questions or need further assistance. See you next time and don't miss to bookmark.