Thermal Radiation Gets Its Name Because __________.
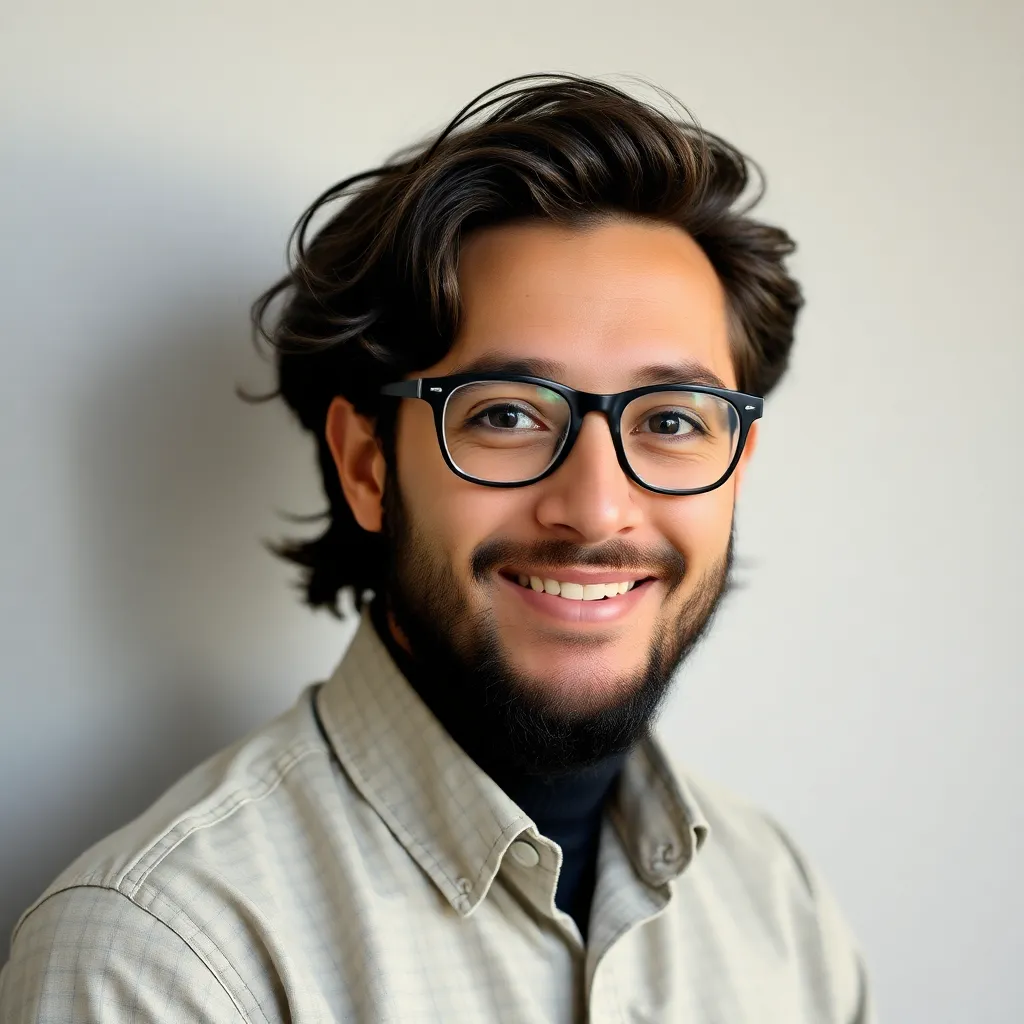
Breaking News Today
Mar 30, 2025 · 6 min read
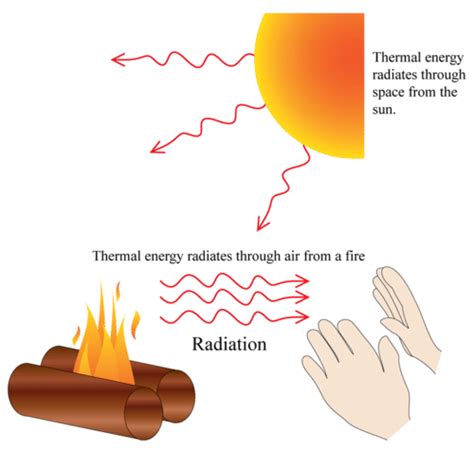
Table of Contents
Thermal Radiation Gets Its Name Because… Heat Transfer!
Thermal radiation gets its name because it's a method of heat transfer that involves the emission of electromagnetic waves. Unlike conduction and convection, which require a medium for heat transfer, thermal radiation can travel through a vacuum. This unique characteristic makes it crucial for understanding various phenomena, from the warmth we feel from the sun to the operation of infrared cameras. Let's delve deeper into the fascinating world of thermal radiation, exploring its nature, mechanisms, and applications.
Understanding the Fundamentals of Thermal Radiation
At the heart of thermal radiation lies the concept of electromagnetic radiation. All objects with a temperature above absolute zero (0 Kelvin or -273.15° Celsius) emit electromagnetic waves. These waves carry energy away from the object, resulting in a loss of heat. The higher the object's temperature, the greater the amount of thermal radiation it emits, and the shorter the wavelengths of the emitted radiation. This relationship is described by Planck's Law, a fundamental equation in quantum mechanics.
Key characteristics of thermal radiation:
- Emission from all matter: Every object, regardless of its material composition, emits thermal radiation.
- Wavelength dependence on temperature: Hotter objects emit radiation at shorter wavelengths, appearing brighter and often changing color. This is evident in the progression from dull red to bright white as an object is heated. Wien's displacement law provides a mathematical description of this relationship.
- Vacuum propagation: Thermal radiation doesn't need a medium to travel; it can propagate through the vacuum of space. This is how the sun's heat reaches Earth.
- Absorption and reflection: Materials interact with thermal radiation differently. Some materials absorb radiation effectively, converting it into heat, while others reflect it. The emissivity of a material describes its ability to emit thermal radiation, while absorptivity describes its ability to absorb it. A perfect blackbody absorbs all incident radiation and emits radiation at the maximum possible rate for its temperature.
The Electromagnetic Spectrum and Thermal Radiation
Thermal radiation occupies a specific portion of the electromagnetic spectrum. While the entire spectrum encompasses various types of radiation, from radio waves to gamma rays, thermal radiation primarily resides in the infrared (IR) region. However, as temperature increases, the emitted radiation can extend into the visible and even ultraviolet regions. This is why incredibly hot objects, like stars, emit visible light.
The intensity of the radiation at each wavelength is crucial in understanding the characteristics of the thermal emission. A plot of intensity versus wavelength is often called a spectral radiance curve. This curve's shape changes depending on the temperature of the emitting body. Higher temperatures lead to curves peaking at shorter wavelengths and higher intensities, reflecting a greater total energy emitted.
Mechanisms of Thermal Radiation Emission
At a microscopic level, thermal radiation arises from the thermal motion of charged particles within a material. These charged particles, including electrons and atomic nuclei, undergo vibrations and oscillations. These accelerated movements generate electromagnetic fields that propagate outwards as electromagnetic waves. The frequency and intensity of these waves depend on the temperature and material properties of the object.
Different materials exhibit varying degrees of emissivity. A blackbody, a theoretical object that perfectly absorbs all incident radiation, serves as a reference point. Real-world materials have emissivities less than one, meaning they emit less thermal radiation than a blackbody at the same temperature. The surface characteristics of the material, such as roughness and color, significantly influence its emissivity. Shiny, polished surfaces generally have lower emissivities than dull, matte surfaces.
The Stefan-Boltzmann Law: Quantifying Thermal Radiation
The Stefan-Boltzmann law provides a quantitative relationship between the total energy radiated by a blackbody and its absolute temperature. The law states that the total power radiated per unit area (P/A) is proportional to the fourth power of the absolute temperature (T):
P/A = σT⁴
Where:
- P is the power radiated
- A is the surface area
- T is the absolute temperature (in Kelvin)
- σ is the Stefan-Boltzmann constant (5.67 x 10⁻⁸ W/m²K⁴)
This law highlights the significant impact of temperature on thermal radiation. A small increase in temperature leads to a substantial increase in the emitted power. This is why even small temperature differences can result in noticeable heat transfer through radiation.
Applications of Thermal Radiation
The principles of thermal radiation find numerous applications across various scientific and engineering disciplines. Let’s explore some prominent examples:
1. Remote Sensing and Thermography: Infrared cameras detect thermal radiation emitted by objects. This technology is widely used in various fields, including:
- Medical diagnosis: Infrared thermography can detect variations in skin temperature, which can indicate underlying medical conditions.
- Building inspection: Identifying areas of heat loss in buildings to improve energy efficiency.
- Industrial monitoring: Detecting overheating components in machinery to prevent equipment failure.
- Night vision: Generating images in low-light conditions by detecting the infrared radiation emitted by objects.
2. Astronomy and Astrophysics: The study of stars and other celestial bodies heavily relies on analyzing the thermal radiation they emit. Astronomers use telescopes equipped with infrared detectors to observe objects that are too cool to emit visible light. The spectral analysis of this radiation provides valuable information about the temperature, composition, and motion of celestial objects.
3. Energy Generation and Transfer: Thermal radiation plays a vital role in energy generation technologies, such as:
- Solar thermal power plants: Concentrating sunlight to heat a fluid, which is then used to generate electricity.
- Radiative heat transfer in furnaces and boilers: Designing efficient heat exchangers requires understanding the radiative heat transfer mechanisms.
4. Environmental Monitoring: Thermal imaging is used to monitor environmental conditions, including:
- Forest fire detection: Early detection of wildfires using infrared sensors mounted on aircraft or satellites.
- Monitoring wildlife: Studying animal behavior and distribution using thermal cameras.
5. Material Science and Engineering: Understanding the thermal radiation properties of materials is crucial in various engineering applications, including:
- Designing thermal insulation: Minimizing heat loss or gain through the use of materials with low emissivity.
- Developing coatings with specific thermal properties: Designing coatings to enhance or reduce the thermal radiation emitted by surfaces.
Beyond the Basics: More Complex Considerations
While the Stefan-Boltzmann law and Planck's law provide fundamental descriptions of thermal radiation, understanding real-world scenarios often requires considering more complex factors. These include:
- Surface properties: The emissivity, absorptivity, reflectivity, and transmissivity of surfaces significantly influence the amount of radiation emitted, absorbed, and reflected.
- Geometry: The shape and orientation of objects influence the radiative heat exchange between them. Consideration of view factors (the fraction of radiation leaving one surface that strikes another) is crucial for accurate modelling.
- Non-uniform temperature distributions: Objects often have non-uniform temperatures, leading to complex patterns of radiation emission. Numerical methods are often required to model these scenarios accurately.
- Participating media: When thermal radiation travels through a medium like air or gas, it can interact with the medium's components, resulting in absorption, scattering, and emission of radiation. This significantly complicates the modelling of radiative heat transfer.
Conclusion
Thermal radiation, named for its role in heat transfer, is a fundamental phenomenon with widespread implications. Its ability to travel through a vacuum, the dependence of its intensity on temperature, and its interaction with matter make it crucial for understanding many natural processes and technological advancements. From medical imaging to astronomy to energy production, thermal radiation plays a key role, highlighting its importance across diverse scientific and engineering disciplines. The continued exploration and refinement of our understanding of thermal radiation will undoubtedly lead to further innovations and breakthroughs in the future. The ongoing development of sophisticated numerical models and experimental techniques continue to improve our predictive capabilities and application of this vital form of heat transfer.
Latest Posts
Latest Posts
-
X Ray Record Image Of The Spinal Cord
Apr 01, 2025
-
Justin Is Upset That He Wasnt Assigned
Apr 01, 2025
-
Which Aspect Of The Definition Of Happiness Gives The Impression
Apr 01, 2025
-
Which Eye Disorders Are Caused By Staphylococcus Aureus
Apr 01, 2025
-
Unit 6 Progress Check Mcq Part C
Apr 01, 2025
Related Post
Thank you for visiting our website which covers about Thermal Radiation Gets Its Name Because __________. . We hope the information provided has been useful to you. Feel free to contact us if you have any questions or need further assistance. See you next time and don't miss to bookmark.